Structural and Mechanical Investigation of Class I Biomimetic Composite Dental Filling by X-ray Computed Tomography, Scanning Electron Microscopy, and Microtensile Bond Strength Testing
Abstract
Nowadays, short fiber-reinforced composites are broadly used in modern restorative dentistry. The tensile strength, Young's modulus, density, and polymerization shrinkage of these materials are developed to mimic the original teeth tissue best. The shrinkage, however, will occur during any polymerization process, which can cause residual stress and gap formation between the tooth and the filling. These can lead to the propagation of cracks, reduction in the adhesive bond strength, or even cause separation in the restoration. During this research, a biomimetic dental restoration was created on a surgically removed third molar with EverX short-fiber reinforced dental composite by bulk-filling technique. And further details about composite resrestorations in biomimetic concept are accessible for you to learn in our course "The Biomimetic concept: composite restoration protocols".
The restored tooth sample was examined by X-ray computed tomography to obtain a 3D image of the whole restoration. The volume of the used adhesive, the material discontinuities, and internal cavities were determined. A cut-out slice was further investigated by scanning electron microscopy to examine the adhesive layer thickness, the gap formation, and the quality of the filling. Finally, microtensile specimens were machined to evaluate the bond strength between the tooth dentin and the filling material.
1 Introduction
Dental science, including the methodology and materials science of tooth restoration, is a dynamically developing field. Thanks to a novel biomimetic approach, the restoration of deep Class I cavities can be filled with shortfiber-reinforced composite resins, preventing significant tooth material removal. Thanks to the low polymerization shrinkage and good translucency of these biomimetic materials, larger cavities (~5 × 5 × 5 mm) can be filled in one step [1]. During the crosslinking of these light-cured short fiber-reinforced composites, polymerization shrinkage is still inevitable, which might lead to gap formation and crack propagation causing a reduction in the bond strength [2]. To characterize these biomimetic restorations, non-destructive and destructive examination methods can be used.
The measurement methods have also changed in recent years with the growing importance of biomedical engineering, where basic engineering assumptions and methods are applied to medical applications so that quantifiable results can be achieved through reproducible practice [3].
Biomimetics is an innovative, multidisciplinary scientific approach, which focuses on biology, engineering, and other sciences, while aiming to develop solutions to a variety of practical problems by modeling biological systems [4]. Every tissue and organ in the oral cavity has its unique properties, which must be considered when creating restorations. In biomimetic dentistry, significant advances have been achieved in the design and regeneration of dental hard and soft tissues [5]. Its aim is to be able to develop a restoration compatible with the original tooth tissue's structural, functional, and biological properties [6].
In a study carried out by Shimada et al. [7], a non-destructive visual examination method called swept-source optical coherence tomography (SS-OCT) was used to observe the cavity adaptation of dental restorations. This non-invasive imagining device does not utilize ionizing radiation; therefore, it is not harmful regarding biological tissues and thus can be used in dental practice. Although, since the SS-OCT method is based on the utilization of optical interference, the size and the material of the observable structures are limited because only the depth penetrated by light can be examined.
During the study, to recreate a similar environment to the dental practice, while establishing structures observable by SS-OCT, the dentin replacement composite was filled in melamine resin with a higher degree of transparency instead of a real tooth.
Another study, by Sadr et al. [8] also used the potential of the SS-OCT technology to examine various dental restoration methods. During the investigation, in addition to creating a 3D image that represents the whole structure after polymerization, 2D real-time videos were also recorded during the light curing. Similar to the previous study, to reach sufficient OCT signal intensity at the bottom of the cavity to examine the gap between the restoration and the cavity floor, a composite mold was used with higher transparency instead of real human molars.
Another non-destructive imaging method to evaluate dental restorations (among many other structures) is the use of micro-computed tomography (µCT). With the help of this method, microstructures, defects, and also differences in density and morphology can be analyzed [9].
The µCT scan uses X-ray to transilluminate objects, allowing the size, homogeneity, and location of each tooth tissue to be observed. The machine examines the whole volume of the sample slice by slice, and at the end of the examination, a 3D image can be obtained [10]. The disadvantage of this method is that it can only be used on removed teeth, since the equipment uses high acceleration voltage X-ray radiation to acquire results with the highest possible resolution. The medical CT equipment has much lower resolution to ensure minimal X-ray radiation [11].
In order to receive 3D images of restored human teeth, the µCT method has to be utilized. During a study by Oglakci et al. [12], different bulk-fill composites and restoration techniques on Class II cavities were evaluated with the beforementioned method. Since a 3D image is received after the examination, the gap formation between the restorative materials and the walls of the cavity could be quantified by image analysis on the measurement of black volumes.
Demirel et al. [13] also examined Class II cavities by micro-computed tomography, five different bulk-fill resin composites were evaluated based on the extent of void formation after light curing.
The effect of different filling techniques on internal void formation in Class I cavities was also examined by Demirel et al. [14] in another study. During sample preparation, the occlusal (chewing) surface was cut to obtain a flat plane before drilling cylindrical cavities, which were filled up later. In total 160 freshly extracted, caries and defect-free human third molars were evaluated by µCT method in the study.
In addition to morphological examination methods, a quasi-static strength-based method called microtensile bond strength (µTBS) test has been one of the most relevant and important testing procedures in the development of dentin adhesive systems. With this testing method, the load capacity of the adhesive bond between the dentin and the filling material can be quantified, giving a basis for the comparison of various adhesive systems, filling materials, and dental procedures. Most previous studies related to dentin adhesive systems utilize the µTBS test to its usefulness and the comparability of its results [15–18].
During this study, a Class I biomimetic dental restoration (Fig. 1) was made by an experienced dentist, simulating a dental procedure used in practice. Compared to conventionally filled restorations, the strength and durability are expected to be more favorable. The fracture toughness and the microstructure of conventional dental fillings significantly differ from those of dentin, causing dissimilarities in the structure. Thus, applying a more dentin-like short fiber reinforced composite with higher fracture toughness may lead to improvement [19, 20]. The aim of this study was to develop and demonstrate a complex method to test the internal integrity and bond strength of dental fillings.
Our primary goal was to test a filling with a non-destructive 3D imaging method, and a destructive, microscopy imaging method before and after microtensile bond strength tests. Our secondary goal was to validate the results of the μCT analysis with electron microscopy images. In addition to the articles mentioned before, it is our goal to validate the results of the µCT analysis with electron microscopy images.
Fig. 1 Schematic figure of a Class I cavity in a molar marked with green
2 Materials and methods
2.1 Materials used in the study
As dentin replacement material, the EverX Posterior (GC Corporation, Tokyo, Japan) shortfiber-reinforced composite was used. The polymerization shrinkage of this material is minor, making it possible to create a gap-free connection, and the glass fibers can prevent the propagation of cracks within the filling [21].
The Estelite Asteria OCE (Tokuyama Dental Corporation Inc., Tokyo, Japan) is an enamel replacement material, which, thanks to its favorable properties, closely resembles the enamel, thus creating an aesthetic restoration.
To promote the crosslinking of the adhesive, a flowable resin, the Clearfil Majesty flow composite was used (Kuraray Co., Ltd., Tokyo, Japan). Since the applied layer of adhesive is thin, and the oxygen located on top of the layer would prevent its polymerization in small volumes, a thicker layer of the latter mentioned composite is applied over the adhesive to avoid this phenomenon.
To ensure the connection between the filling material and the tooth, Clearfil SE Protect (Kuraray Co., Ltd., Tokyo, Japan), a light-cured, self-etch two-steps adhesive system was applied. Its special feature is that it contains functional monomers, like MDPB with antibacterial cavity-clearing properties and 10-MDP which creates a chemical bond to the hard tissues of the tooth.
2.2 Method of dental restoration
During this current research, one otherwise healthy third molar was removed for orthodontic reasons. To obtain the ideal filling, it is essential to adhere to the exact timeframes during the procedure.
In the first step of the preparation, a 4 × 4 × 4 mm Class I cavity was created by an 850018SC (NTI-Kahla GmbH, Kahla, Germany) instrument in a Ti Max Z95L (NSK Corporation, Ann Arbor, MI, USA) electric dental handpiece at 40000 rpm with water cooling in an extracted third molar. This was followed by 10 seconds of sandblasting on 5 bar pressure with 27 μm Al2O3 particles from a distance of 2−4 mm. The next step was selective etching of the enamel with Blue Etch gel (PPH CERKAMED Wojciech Pawlowski, Stalowa Wola, Poland) orthophosphoric acid for 20 seconds, then 10 seconds of rinsing with water and drying with compressed air on 6 bar pressure. The cavity was then rubbed with Clearfil SE Protect Primer for 30 seconds to penetrate into the dentin, then blown by compressed air for 30 seconds to evaporate all the solvent from the primer. The application of the Clearfil SE Protect Adhesive was the next step, which was only thinned with a microbrush and the excess was removed with suction. The adhesive was cured with a VRN VAFU LED lamp (Guilin Veirun Medical Technology Co., Ltd., Guangxi, China) for 20 s, at 476 nm, and 1400 mW/cm2.
The oxygen inhibits the polymerization up to a certain thickness (10−20 µm in case of adhesive resins [22, 23]). An additional thin composite layer is required to counteract the polymerization inhibiting effect of oxygen. Clearfil Majesty Flow composite was applied on top of the bond layer at a thickness of 0.5 mm. Lastly, the structure was cured with a polymerization lamp for 20 seconds.
As for creating the restoration, a bulk filling method was used in this current study. After the preparation, the cavity was filled with the EverX Posterior dentin replacement material and crosslinked with polymerization light two times for 20 seconds. The missing enamel was restored with Asteria Estelite OCE composite, in several layers, cusp by cusp. Each layer was polymerized separately for 20 seconds.
2.3 Examination methods
After the preparation of the fillings, it is recommended to use non-destructive material testing methods to examine the restored teeth prepared by dentists, as the other testing methods require the destruction of the samples. This study was performed using a YXLON FF35 dual-tube computed tomography equipment (YXLON International GmbH, Hamburg, Germany) with a 3.9-megapixel detector, a resolution of 0.6 µm in 3D and 0.15 µm in 2D, and a metrological measurement error of maximum 3.2 µm. The samples were reconstructed and evaluated using VGSTUDIO MAX 3.4 software [24].
To further examine the internal structure of the filled teeth, and to perform the microtensile bond strength test, proper sample preparation was necessary (Fig. 2). As the first step, the tooth was attached to a Ø20 mm diameter Duracryl Plus two-component resin cylinder (SpofaDental Inc., Jičín, Czech Republic) with StickyStar (S & S Scheftner GmbH, Mainz, Germany) dental wax.
The tooth was then cut with a Buehler IsoMet 1000 diamond disc cutter (Buehler Ltd., Lake Bluff, IL, USA) at the center of the tooth, perpendicular to the occlusal plane, opening the pulp cavity, which was filled with conventional dental composite then crosslinked. After this, the second cut was perpendicular to the previous cut, this way, ~2 mm thin slices were obtained (Fig. 2(d)), which were examined later by scanning electronic microscopy (SEM).
For the µTBS tests, these slices were further cut to rectangle-based blocks (Fig. 2(e)). After the preparation of the slices, scanning electronic microscopy (SEM) examination was used, where the goldplated sample was scanned by a focused electron beam.
The specimens were investigated with a Zeiss EVO MA10 scanning electron microscope (Carl Zeiss AG, Jena, Germany) at 20 kV accelerating voltage to investigate the layers of the filing and possible gaps created by polymerization shrinkage in 2D. Seconder electron (SE) detector was used for the characterization of the layers and for the investigation of the fractured surfaces. For further analysis, backscatter electron (BSE) detector was used on the fractured dental filling.
With the prepared rectangle-based block specimens, the µTBS examinations were performed by an Instron 5965 electromechanical material testing device (Instron, Norwood, MA, USA). The machine was equipped with a 5 kN load cell, and the measurements were implemented with a cross-head speed of 1 mm/min. The microtensile bond strength test were carried out with specially designed jigs to further improve reproducibility (Fig. 3). This type of jig is used for the microtensile testing of various materials, since the aligning pins restrict the torsonal and bending loads; therefore, even brittle materials can be loaded with pure tension.
After the µTBS tests, the fractured surface areas were investigated by SEM. By this examination method the exact location of fracture can be determined.
Fig. 2 Preparation steps for the morphological and mechanical examinations: a) attachment of the tooth for cutting, b) opening the pulp cavity, c) marking the cutting lines for the slices, d) cut slice and e) cut µTBS testing specimens
Fig. 3 Jig used for the microtensile bond strength tests
3 Results and discussion
3.1 Results of the µCT analysis
In the images obtained from the tests, the different density layers show different greyness values, so the individual layers can be clearly distinguished. By examining individual layers of the resulting 3D model, gaps, separations and inclusions can be observed.
The µCT image slice (Fig. 4) shows the layers of the restored tooth, and it can be observed that in the case of direct bulk fillings, there is a material with air-like density at the bottom of the cavity and along the wall, which needs to be examined using other imaging techniques.
Fig. 4 µCT image of the direct bulk filled tooth
In order to investigate the boundary layers between each material and tissue, an edge detection test was run. This determined the volume of the delimited parts and their location, which can be observed in Fig. 5. The volume of the filling and the extent of the boundary layer was determined as 24.6 mm3 and 0.80 mm3, respectively.
Fig. 5 Visual simulation run to examine boundary layers
3.2 Results of the SEM examinations
The SEM images confirm the results of the CT scan. In Fig. 6, it can be observed that the material at the bottom of the filling, which resembles the density of air on the CT scan, appears to be the adhesive used for the filling. For confirmation of the assumption, the extent of the adhesive was observed with CT imaging.
The average adhesive layer thickness is quantifiable with the SEM image of the cross-section of the restoration. The beforementioned numerical data was calculated with JMicroVision 1.2.7 image analysis software [25]. The average thickness was 38.48 ± 30.25 µm. Fig. 6. shows the reason behind the relatively significant standard deviation, as the adhesive is not distributed uniformly on the bottom of the cavity.
The results of the SEM examinations after the µTBS tests can be seen in Fig. 7. It is clearly visible, that the failure occurred on the tooth-filling boundary layer, as the fracture surface is relatively smooth.
The fracture surface has brittle characteristics at the boundary of the dentin and the filling; however, as Fig. 7 shows, locally, at a well-defined area, the fracture occurred within the adhesive (marked with arrows).
Fig. 6 SEM image of the direct bulk-filled restoration
Fig. 7 SEM images of the surface areas of the fracture after the µTBS test: a) SE detector image of the molar side, b) SE detector image of the filling side, and c) BSE detector image of the tooth side
3.3 Results of the microtensile bond strength tests
From the investigated tooth, a total of five tensile specimens were cut out. The average and standard deviation of the measured microtensile bond strength was 28.6 ± 3.8 MPa.
The maximum bond strength was 37.4 MPa which is approaching the strength of the dentin-enamel junction (~51.1 MPa [26]). The bond strength can be further improved with levelling the bottom of the cavity flatter, and applying the adhesive evenly, with quasi-constant thickness.
You have the opportunity to gather more in-depth information about biomimetic approach in our course "Indirect restoration in the biomimetic concept".
4 Conclusion
The research objectives have been successfully achieved by conducting and evaluating material analysis of a biomimetic restoration. The presented methods can be applied onto any dental restoration. It can be stated that the µCT scan is suitable for non-destructive microstructural analysis of dental samples. Still, software evaluations are complex due to the small size of the sample and the roughness of the internal surfaces. The greyness value of the adhesive is very close to that of air, so additional measurements are needed to assess gaps and separations using different methods. However, 3D imaging provides superior information about the restoration, compared to any 2D imaging or mechanical testing.
Acknowledgements
The research activities described in this paper were approved by the Hungarian Medical Research Council (TUKEB), permission No. IV/8518-1/2021/EKU. The publication of the work reported herein has been supported by EHK at BME. Project no. TKP-6-6/PALY-2021 has been implemented with the support provided by the Ministry of Culture and Innovation of Hungary from the National Research, Development and Innovation Fund, financed under the TKP2021-NVA funding scheme.
List of authors:
Levente Borhy, Péter Zoltán Farkas, Borbála Leveles, Alexandra Kemény, András Volom, Balázs Varbai
References
Tsujimoto, A., Barkmeier, W. W., Takamizawa, T., Latta, M. A., Miyazaki, M. "Bonding performance and interfacial characteristics of short fiber-reinforced resin composite in comparison with other composite restoratives", European Journal of Oral Sciences, 124(3), pp. 301–308, 2016.
Yadav, R., Kumar, M. "Dental restorative composite materials: A review", Journal of Oral Biosciences, 61(2), pp. 78–83, 2019.
Han, S.-H., Sadr, A., Tagami, J., Park, S.-H. "Non-destructive evaluation of an internal adaptation of resin composite restoration with swept-source optical coherence tomography and micro-CT", Dental Materials, 32(1), pp. e1–e7, 2016.
Salim, F. M. "Tribological and mechanical characteristics of dental fillings nanocomposites", Energy Procedia, 157, pp. 512–521, 2019.
Upadhyay, A., Pillai, S., Khayambashi, P., Sabri, H., Lee, K. T., Tarar, M., Zhou, S., Harb, I., Tran, S. D. "Biomimetic aspects of oral and dentofacial regeneration", Biomimetics, 5(4), 51, 2020.
Basheer, N., Madhubala, M. M., Mahalaxmi, S. "Future Perspectives of Biomimetics in Restorative Dentistry", Journal of Pharmaceutical Research International, 32(25), pp. 19–28, 2020.
Shimada, Y., Sadr, A., Nazari, A., Nakagawa, H., Otsuki, M., Tagami, J., Sumi, Y. "3D evaluation of composite resin restoration at practical training using swept-source optical coherence tomography (SS-OCT)", Dental Materials Journal, 31(3), 409–417, 2012.
Sadr, A., Bakhtiari, B., Hayashi, J., Luong, M. N., Chen, Y.-W., Chyz, G., Chan, D., Tagami, J. "Effects of fiber reinforcement on adaptation and bond strength of a bulk-fill composite in deep preparations", Dental Materials, 36(4), pp. 527–534, 2020.
Ghavami-Lahiji, M., Davalloo, R. T., Tajziehchi, G., Shams, P. "Micro-computed tomography in preventive and restorative dental research: A review", Imaging Science in Dentistry, 51(4), pp. 341–350, 2021.
Hayashi, J., Shimada, Y., Tagami, J., Sumi, Y., Sadr, A. "Real-Time Imaging of Gap Progress during and after Composite Polymerization", Journal of Dental Research, 96(9), pp. 992–998, 2017.
Hirata, R., Pacheco, R. R., Caceres, E., Janal, M. N., Romero, M. F., Giannini, M., Coelho, P. G., Rueggeberg, F. A. "Effect of sonic resin composite delivery on void formation assessed by micro-computed tomography", Operative Dentistry, 43(2), pp. 144–150, 2018.
Oglakci, B., Kazak, M., Donmez, N., Dalkilic, E. E., Koymen, S. S. "The use of a liner under different bulk-fill resin composites: 3D GAP formation analysis by x-ray microcomputed tomography", Journal of Applied Oral Science, 28, e20190042, 2020.
Demirel, G., Baltacıoğlu, İ. H., Kolsuz, M. E., Ocak, M., Orhan, K. "Micro-computed tomography evaluation of internal void formation of bulk-fill resin composites in Class II restorations", Polymer Composites, 40(8), pp. 2984–2992, 2019.
Demirel, G., Orhan, A. I., Irmak, Ö., Aydin, F., Buyuksungur, A., Bilecenoğlu, B., Orhan, K. "Micro-computed tomographic evaluation of the effects of pre-heating and sonic delivery on the internal void formation of bulk-fill composites", Dental Materials Journal, 40(2), pp. 525–531, 2021.
Porto, I. C. C. M., Nascimento, T. G., Oliveira, J. M. S., Freitas, P. H., Haimeur, A., França, R. "Use of polyphenols as a strategy to prevent bond degradation in the dentin–resin interface", European Journal of Oral Sciences, 126(2), pp. 146–158, 2018.
van den Breemer, C. R. G., Özcan, M., Cune, M. S., Almeida Ayres, A. P., Van Meerbeek, B., Gresnigt, M. M. M. "Effect of immediate dentin sealing and surface conditioning on the microtensile bond strength of resin-based composite to dentin", Operative Dentistry, 44(6), pp. E289–E298, 2019.
Sano, H., Chowdhury, A. F. M. A., Saikaew, P., Matsumoto, M., Hoshika, S., Yamauti, M. "The microtensile bond strength test: Its historical background and application to bond testing", Japanese Dental Science Review, 56(1), pp. 24–31, 2020.
Alhenaki, A. M., Attar, E. A., Alshahrani, A., Farooq, I., Vohra, F., Abduljabbar, T. "Dentin Bond Integrity of Filled and Unfilled Resin Adhesive Enhanced with Silica Nanoparticles—An SEM, EDX, Micro-Raman, FTIR and Micro-Tensile Bond Strength Study", Polymers, 13(7), 1093, 2021.
Lassila, L., Säilynoja, E., Prinssi, R., Vallittu, P. K., Garoushi, S. "Fracture behavior of Bi-structure fiber-reinforced composite restorations", Journal of the Mechanical Behavior of Biomedical Materials, 101, 103444, 2020.
Lassila, L., Keulemans, F., Vallittu, P. K., Garoushi, S. "Characterization of restorative short-fiber reinforced dental composites", Dental Materials Journal, 39(6), pp. 992–999, 2020.
Tanner, J., Tolvanen, M., Garoushi, S., Säilynoja, E. "Clinical Evaluation of Fiber-Reinforced Composite Restorations in Posterior Teeth - Results of 2.5 Year Follow-up", The Open Dentistry Journal, 12, pp. 476–485, 2018.
Van Meerbeek, B., Yoshihara, K., Van Landuyt, K., Yoshida, Y., Peumans, M. "From Buonocore's Pioneering Acid-Etch Technique to Self-Adhering Restoratives. A Status Perspective of Rapidly Advancing Dental Adhesive Technology", The Journal of Adhesive Dentistry, 22(1), pp. 7–34, 2020.
Rueggeberg, F. A., Margeson, D. H. "The Effect of Oxygen Inhibition on an Unfilled/Filled Composite System", Journal of Dental Research, 69(10), pp. 1652–1658, 1990.
VolumeGraphics "VGSTUDIO MAX 3.4 software", [computer program]
Roduit, N. "JMicroVision 1.2.7 image analysis software", [computer program]
Urabe, I., Nakajima, S., Sano, H., Tagami, J. "Physical properties of the dentin-enamel junction region", American Journal of Dentistry, 13(3), pp. 129–135, 2000.
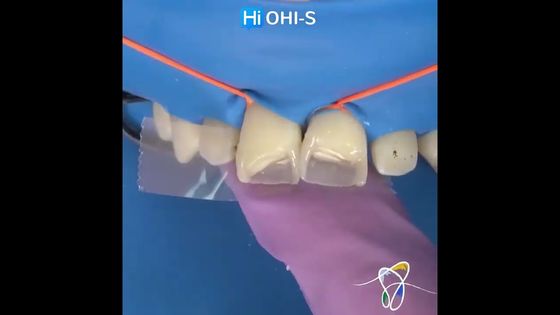
Video
OHI-S
02 April 2024
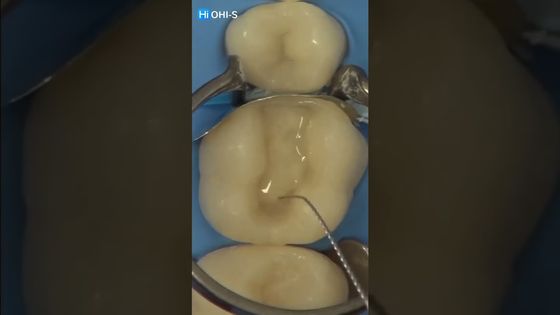
Video
OHI-S
16 January 2024
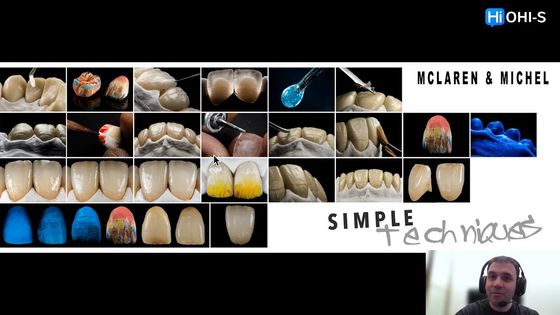
Video
OHI-S
21 November 2023