Light and Viscosity Effects on the Curing Potential of Bulk‑Fill Composites Placed in Deep Cavities
Abstract
To determine the infuence of light curing units (LCUs) and material viscosity on the degree of conversion (DC) of bulk-fill (BF) resin-based composites (RBCs) placed in deep cavity preparations. Four LCUs were tested: Valo cordless, Bluephase-G2, Poly wireless and Radii-cal. Light irradiance was determined at 0 mm and 6 mm distance to the reading sensor. The following RBCs were considered: Filtek BF, Filtek BF Flow, Opus BF, Opus BF Flow, Tetric N-Ceram BF and Surefl SDR Flow. Sirius-Z was used with the incremental technique. Additional details about composite materials and direct restoration techniques are accessible for you to learn on our website in Direct Restoration section.
DC (n=3) was evaluated by spectroscopy both at top and bottom regions of deep preparations with 6 mm depth. The data were submitted to ANOVA and Tukey’s test (α=0.05). Pearson’s correlation (95%) was used to verify the relation between the LCUs and the curing potential of RBCs. The DC at 6 mm depth was reduced when Opus BF, Opus BF Flow and Tetric N-Ceram BF were activated with Radii-cal. There was a positive correlation between the LCU irradiance and the bottom/top conversion ratios. The materials’ viscosities did not afect the curing potential. Bulk-fill composites did not present higher curing potential than the conventional composite used with the incremental technique; the most important aspect of the LCU was the irradiance ratio; and the materials’ viscosity did not afect the curing potential as a function of depth. Radii-cal negatively impacted the degree of conversion at 6 mm depth for most bulk-fill resin composites. Depending on the brand, bulk-fll composites may present reduced curing potential due to the light source when placed in deep cavities. Dentists should avoid LCU with acrylic tips to photoactivate bulk-fill resin-based composites.
Introduction
The bulk-fill resin-based composites (RBCs) were developed to optimize clinical time and minimizing adversities from the incremental technique [1]. Available in regular and fowable viscosities, they are not necessarily a great technological leap [2], but the result of a series of facts that ended up converging on the possibility of using this class of materials in clinical situations [3–6].
Due to the placement of thicker increments, the need for increased curing light transmittance becomes crucial for optimal polymerization and ultimately the clinical success of bulk-fill RBCs. It is known that the use of light curing units (LCUs) that have high radiant exitance and that emit a wavelength within the absorption spectrum of the photoinitiators are fundamental to initiate, and provide sufcient polymerization [7–10]. Many LCUs are available in the dental market, varying in terms of the power supply (mains electric, or battery operated), active area of light curing tip, their ergonomic and optical design, i.e. light transmitted through fber optic bundles (“pistol”) compared with the LED diode(s) positioned within the head of the light curing tip (“stick”), activation mode, and spectral irradiance (diode type(s), wavelength(s) and power), and, obviously, the cost.
Previous studies have supported manufacturers’ assertions that bulk-fill RBCs can achieve adequate depth of cure if an adequate LCU radiant emittance is used [7, 11]. On the other hand, some investigations have reported decreased degree of conversion (DC) towards the lower surfaces of bulk-fill RBCs using manufacturers’ recommended curing exposure times [12, 13]. In addition to these contradictions, it is essential to consider the fact that in vitro studies carried out with bulk-fill RBCs commonly employ ideal laboratory conditions with 4 mm depth moulds [3, 7, 14, 15].
This is not according to many clinical situations in which the curing tip to material surface is usually greater than 0 mm distance and certainly more than 4 mm from the bottom of the cavity preparation, e.g. the gingival margin of a class II cavity, which is the point of failure of many posterior RBC-tooth restorations. Since manufacturers ofer bulk-fill materials in a variety of compositions, a further clinical question remains the efect of material viscosity on polymerization at increased depths [7].
In view of the existing doubts so far, the main objective of this study was to determine the polymerization characteristics of bulk-fill RBCs in deep material layers. Specifcally, the current aims were to (a) characterize the polymerization ability of bulk-fill RBCs when used in deep preparations, (b) determine the efect of the LCU parameters’ of irradiance on the polymerization ability of bulk-fill RBCs; and (c) analyze whether the viscosity of the bulk-fill RBC infuences the polymerization ability.
The hypotheses of the current study were, that:
bulk-fill composites would present higher curing potential than a conventional composite used with the incremental technique regardless of the LCU used;
the influence of the LCU on the polymerization capacity at great depths would be dependent on the tip irradiance;
the low-viscosity bulk-fill RBC would have greater polymerization ability in deep preparations when compared with the higher viscosity materials.
Materials and methods
Materials
Four light-emitting diodes (LED) LCUs were investigated: two single diode (so-called, “monowave”) LCU types: Poly wireless (Kavo Kerr, Joinville, SC, Brazil) and Radii-cal (SDI, Victoria, Australia), and two multiple diode (so-called, “polywave”) LCU types; Bluephase G2 (‘high power’ mode; Ivoclar Vivadent, Schaan, Liechtenstein), and Valo cordless (‘standard mode’; Ultradent, South Jordan, UT, USA) (Table 1).
Table 1 Specifcations and irradiance values of light curing units
Seven commercially available RBCs were tested: a conventional, non-bulk-fill material (control; tested by the incremental insertion technique—Siriuz-Z (DFL, RJ, Brazil); three bulk-fill RBCs of paste-like viscosity—Filtek Bulk Fill (3M, St Paul, MN, USA), Opus Bulk-fll APS (FGM, Joinville, SC, Brazil) and Tetric N-Ceram Bulk Fill (Ivoclar Vivadent), and three bulk-fll RBCs with lower viscosity—Filtek Bulk Fill Flow (3M), Opus Bulk-fll Flow APS (FGM), SureFil SDR Flow (Dentsply, Milford, DE, USA). Table 2 shows the RBCs specifcations, according to manufacturer’s information.
Table 2 Specifcations of investigated materials (manufacturer’ information)
Methods
Light irradiance (mW/cm2) analysis
The internal diameter of each LCU tip was measured with a digital caliper (Mitutoyo, Japan) to calculate the efective tip area of each LCU. The power (mW) of all LCUs was measured using a power meter (Ophir 10A-V2-SH, Ophir Optronics, Har-Hotzvim, Jerusalem, Israel) connected to a NOVA microprocessor (Ophir Optronics) (Fig. 1). With these data, it was possible to determine the irradiance for each LCU. For each LCU, irradiance readings were performed with the light tip juxtaposed to the power meter (0 mm) and also 6 mm from the sensor surface. The ratio between 6 and 0 mm depths was calculated to estimate light collimation and was named as “light irradiance ratio”.
All procedures of power measurement were also performed trough a standardized area, to simulate the conditions used in the Fourier-transformed infrared (FTIR) spectroscopy measurements, using a black tape with a 5 mm diameter hole.
Fig. 1 Sensor “cap” was removed (A) and it was possible to observe the 20 mm diameter sensor (B). This allowed the positioning of the same molds used for the FTIR measurements (C) and also direct contact of all light tips (D)
Degree of conversion
The DC was determined by FTIR (n = 5), using a spectrometer equipped with an attenuated total refectance (ATR) diamond device (Brüker Optics, Ettlingen, Germany). To evaluate the DC close to the LCU tip, a black-color plastic mold with 5 mm in diameter and 0.1 mm in thickness was used. The mold was positioned directly onto the diamond ATR crystal, followed by the insertion of the RBC in a single increment. A transparent polyester strip was then positioned on the RBC increment and the excess was removed by hand pressing a glass slide over this set. The DC at the top area was also used as way to estimate the highest DC for the LCUs and materials tested without the infuence of material thickness.
To evaluate the DC at 6 mm depth, a black-color plastic mold, 5 mm diameter, 6 mm thickness, demarcated at 2, 4 and 6 mm, was used to guide the insertion of the increments. The matrix was positioned directly on the diamond ATR crystal followed by insertion of the increments according to the commonly used techniques: Incremental (control): Sirius-Z RBC was used in 3 increments of 2 mm thickness, always followed by the photoactivation.
Bulk-fll RBCs of low-viscosity associated with conventional RBC as a cover: the low-viscosity bulk-fll RBC was used up to the 4 mm mark, when the photoactivation process was carried out. Over this material, one more increment (2 mm in thickness) was inserted with conventional RBC. To reduce variability, the same RBC employed in the incremental technique was used (Sirius-Z).
Bulk-fll RBCs of regular-viscosity: the regular-viscosity bulk-fll RBC of was used up to the mark of 4 mm, when the photoactivation process was carried out. Over this increment, one additional increment with 2 mm thickness was inserted with the same material and photoactivated. The photoactivation protocols followed the respective manufacturers’ recommendation.
One split-rubber-based matrix used to surround the plastic matrix and avoid light exposure on the side areas as well as refection from the metallic parts from the ART accessory, which might otherwise overestimate DC. The FTIR spectra were collected from the uncured composite and also immediately after photoactivation of each increment. Spectra were obtained with 32 scans and 4 cm resolution−1 of the uncured and cured RBCs. The interval between 1800 and 1600 cm−1 was considered for the observation of the signals in 1608 and 1638 cm−1, corresponding to the aromatic phenyl groups of bisphenol A and aliphatic functional groups of the methacrylate molecules. The DC was calculated using the ratio between the signal height at 1638 cm−1 and at 1608 cm−1 of the cured and uncured RBCs according to the equation:
Statistical analysis
To verify the infuence of the LCUs on the DC in the top and bottom regions, multiple 2-way ANOVA followed by the Tukey’s test (α=0.05) were applied. Independent analyses were performed for each material.
Pearson’s correlation (95%) was used to verify the possibility of a relation between the LCUs irradiance conditions and the polymerization properties of RBCs. The following relations were tested for each resin composite and also with the aggregated values (average DC from all materials tested): direct irradiance versus DC at the top; direct irradiance versus DC at the bottom; 6 mm irradiance versus DC at the top; 6 mm irradiance versus DC at the bottom; irradiance ratio versus DC ration.
To determine the infuence of the viscosity of RBCs on DC in the top and bottom regions, the values obtained with LCU (Valo cordless) were considered and a 3-way ANOVA was conducted and followed by Tukey’s test (α=0.05).
Results
The characterization of the LCUs is detailed in Table 1. Radii-cal showed the most signifcant decrease in irradiance at 6 mm depth compared to the top, maintaining only 43% of the original energy delivered. For the other LCUs, this ratio was 61%, 71% and 75% for Poly wireless, Valo cordless and Bluephase G2, respectively.
When the light area was standardized, only Radii-Cal readings taken at 6 mm depth were lower than those at 0 mm distance. Considering the 2-way ANOVA, the factor LCU afected the DC, with the exception of the RBCs, Filtek BF Flow and Surefl SDR Flow.
Figure 2 shows both the DC mean values obtained 0.1 mm from the top surface and also the cumulative values (after 2 exposures for the bulk-fll materials and 3 for the conventional one) observed on the 6 mm depth. Table 3 shows the isolated and grouped main values of DC at 0.1 and 6 mm regions and the ratio based on the diferent RBCs and LCUs tested. In general, it was verifed that, the LCU exerted low infuence on the DC at 0.1 mm depth. When considering the 6 mm depth, Radii-cal promoted lower values than the other LCUs when Opus BF, Opus BF Flow and Tetric N-Ceram BF were analyzed. By gathering 0.1 and 6 mm depth values, Radii-cal showed statistically lower DC mean values for almost all the tested RBCs, with the exception of Filtek BF Flow and Surefl SDR Flow. Regarding the infuence of the region (0.1 and 6 mm depth), only Surefl SDR Flow did not show statistical diference among the LCUs. For the other bulk-fll RBCs, the 6 mm depth region always showed lower mean values than at 0.1 mm. Conversely, Sirius-Z, applied with the incremental technique showed an inverse result, being the DC at 6 mm depth higher that at 0.1 mm. When gathered, Sirius-Z and Surefl SDR Flow approached 100% top–bottom ratio.
Table 4 shows the correlation coefcients for the comparisons between irradiance conditions and curing properties. The analyses were performed both separately for each material and also with the materials’ aggregated values. It is possible to verify that the correlation between the irradiance ratio—the irradiance value obtained with the 6 mm spacer divided by the irradiance value obtained from the directly measurements on the power meter sensor—and the degree of conversion ratio (DC at the bottom region/DC at the top region) presented the highest correlation.
The efect of material’s brand, viscosity and depth on the DC values can be seen in Table 5. It is possible to observe that all the three factors signifcantly afected the DC, without interactions among them. With regard to materials’ brand, Opus BF produced higher DC values and bottom/top ratios than Filtek BF. The more-viscous materials produced higher DC than the fowable ones but the bottom/top ratios were similar. The DC obtained at the bottom region was statistically lower than those found in the top region, regardless of materials’ brands and viscosities.
Fig. 2 DC values obtained on the subsurface (0.1 mm) and also the cumulative values observed on the 6 mm depth (after 2 exposures for the bulk-fill materials and 3 for the conventional one)
Table 3 Infuence of light curing unit on the degree conversion as a function of depth for each material tested
Table 4 Correlation coefcients for the comparisons between irradiance conditions and curing properties
Table 5 Degree of conversion as a function of depth, materials’ brand and viscosityThere are further details about deep tooth cavities treatment that you can gain in our course "The Biomimetic concept: composite restoration protocols".
Discussion
Most previous studies that analyze the curing potential of bulk-fll RBCs tend to determine the depth of cure using 4 mm thick increments [3, 7, 14, 15]. However, in clinical situations, the photoactivation process of bulk-fll RBCs could represent a challenge when deeper cavities are considered, as the light has to be transmitted over thicker increments compared with that of more traditional techniques. Therefore, the current study simulated preparations with 6 mm depth that were flled with commercially available bulk-fll RBCs light cured by four LCUs and tested them in diferent situations. As some bulk-fll composites were dependent on the LCU tested and the conventional composite—based on 2 mm increment – was not, the frst research hypothesis was rejected.
Although the LCUs did not afect the DC close to the surface, they did infuence those readings taken at 6 mm depth for Opus BF, Opus BF Flow and Tetric BF. Opus BF Flow showed lower conversion at 6 mm depth when the single wavelength band-based LCUs were used. This fnding could be associated with the fact that this RBC presents a combination of diferent photoinitiators. According to the manufacturer, Opus BF and Opus BF Flow have reduced amount of CQ in their formulations and the addition of an “Advanced Polymerization System (APS)” (a given name by the manufacturer, who detains the patent of this product), which interacts with the traditional system and amplifes the polymerization capacity of RBCs. However, and in contrast to the ‘fowable’ RBC, the depth of cure of the more-viscous material was reduced only with Radii-cal and not for Poly wireless. Therefore, the reduced DC promoted by Radiical is likely to be associated with a high dispersion of light than the fact that this LCU does not emit a second peak of light. This statement can be supported by the results found with Tetric N-Ceram BF that also has an alternative initiator system.
Tetric N-Ceram BF has in its composition, “Ivocerin”, an alternative photoinitiator based on germanium salt derivative and sensitive to wavelengths of violet-blue light with the maximum peak of absorption around 410 nm [4]. When the Tetric N-Ceram BF was placed as a 4 mm thick increment and light-cured, the DC mean values were lower than 50% for all LCUs tested, but after the insertion of the second increment and the additional exposure to light, the DC mean values increased and the results were statistically similar for the top region—with the exception of those samples activated with Radii-cal, which produced a top–bottom ration lower than 80%. In contrast, Poly wireless is also a single wavelength band LCU—a contradictory term for a single diode LCU—and produced satisfactory conversion on the bottom region, being not diferent than the multi-wave LCUs Valo or Bluephase. In other words, it seems that the fnal conversion at the bottom region is a fact related to the ratio between the light irradiance close to the surface to that at 6 mm depth than the number of peaks emitted by LCU. Indeed, the need for alternative photoinitiators to be sensitized by the violet spectrum has been previously reported [16]. The violet spectrum is less likely to reach greater depths due to the absorption of most of the violet light in the upper layers of the RBCs [17]. Another explanation for the lack of efectiveness of violet light is due to the relation between the wavelength of light and the dimensions of the inorganic fller particles. According to the Rayleigh-scattering law, fller particles are more likely to scatter shorter wavelengths and therefore the violet spectrum sufers greater light attenuation when compared with the blue spectrum [18]. A systematic review concluded that the multi-wave LCUs are useful but not essential for activation commercially available RBCs containing alternative photoinitiators [19]. Like that, the activation of the alternative photoinitiators will be dependent on the total irradiance emission and the lower irradiance loss of the LCUs.
Sirius-Z was tested as a control group as a conventional material that required an incremental placement technique in 2 mm thicknesses, and its depth of cure was not afected by the LCUs tested in the current investigation. This finding is explained by the fact that the 2 mm thick increments and light exposures were overlapped with a longer exposure time and consequent increase of the fnal DC. In this way, it was possible to achieve a top–bottom ratio close to 100%. The efect of such light exposure “overlapping” is also possible to see with all bulk-fll composites as there is a clear trend of DC increase at the bottom part when the second increment is placed (Fig. 1).
SureFil SDR Flow was not infuenced by the diferent LCUs tested. Considering the DC at the bottom region after the second increment, it did not present signifcant statistical diferences in the DC mean values in relation to the top region and it showed a top–bottom ratio close to 100%. The efciency of the polymerization at great depths of SureFil SDR Flow should be attributed to its higher translucency, which increases the transmission of light through the material [20]. In addition, the presence of a photo reactive group of urethane-based methacrylate monomer is capable of interacting with the CQ, allowing an increase in the DC. Although it exhibits 68% by weight of fller content, which could increase its viscosity and negatively infuence the results of DC and polymerization depth, the low viscosity of SureFil SDR Flow is due to the presence of TEGDMA as the diluent monomer. The absence of strong intermolecular secondary interactions, such as hydrogen bonds, in addition to the low viscosity and high fexibility, gives to TEGDMA high monomeric conversion values, and is a strategy to increase the DC [21].
The second objective of the study was to determine the efect of the LCU irradiance parameters on the polymerization ability of bulk-fll RBCs. The second research hypothesis, which considered that the infuence of the LCUs on the polymerization capacity at increased depths would be dependent on the irradiance directly emitted by the LCU output, was rejected, both by the statistical analyzes of each material and also by the Pearson’s correlation (Table 4). Indeed, the LCU’s irradiance ratio presented the highest correlation with the depth of cure. As the distance afects the amount of light that arrives at the RBC, the specimens were activated at a distance of 6 mm to reproduce a clinical situation of deep preparation and the light irradiance was checked with the light-curing tip positioned close to the power meter sensor as well as at 6 mm distance [22]. In this scenario, Bluephase G2, Valo cordless and Poly wireless presented top–bottom ratios of 75%, 71% and 61%, respectively, and Radii-cal only 43%. The large reduction irradiance of the Radii-Cal in comparison with the others LCUs is probably related to the acrylic-based lens used in its tip which interferes in the light passage—which may be related to the refractive index or degree of translucency of the material used in the tip—and consequent decrease of the irradiance.
The infuence of material viscosity on DC and depth of cure was evaluated for Filtek and Opus RBCs that are commercially available in two viscosities. The DC mean values were statistically higher for the more-viscous materials and the bottom-to-top ratio was similar for both, rejecting the third hypothesis. It was expected that the low-viscosity bulk-fll RBCs would have a greater capacity of polymerization in deep preparations than the regular-viscosity ones due to the greater mobility of monomers in the less viscous media and the fact that the increased amount of fller particles hinders the passage of light through the composite [23]. The current outcomes might be justifed by the diferent photoactivation times used for Opus BF and Opus BF Flow RBCs, being 30 and 20 s, respectively. The low-viscosity of Filtek BF Flow can be also related to the presence of procrylat (2,2-bis[4-(3- methacryloxypropoxy)phenyl]propane), UDMA and Bis-EMA in its organic matrix. Although Bis-EMA is an analogue to Bis-GMA and has a high molecular weight, the absence of two hydroxyl groups is responsible for its lower viscosity and higher conversion [21]. The polymers formed from Bis-EMA and modifed-urethane monomers with reduced viscosity tend to produce high DC values when compared to the typical Bis-GMA/TEGDMA resin matrices. However, the presence of higher molecular weight monomers, such as Bis-GMA, Bis-EMA and UDMA, may have hampered the mobility of reactive species at greater depths and infuenced the fnal DC of the composite.
In addition, because of the low and inherent mechanical properties of the low-viscosity RBCs, the restorative technique requires a fnal 2 mm thick layer with a more resistant and viscous RBC to obtain higher resistance to wear [24]. Then, the second increment of the low-viscosity RBCs was performed with conventional RBC and may have infuenced the low fnal DC mean values in relation to the regular-viscosity RBCs. Another explanation is the fact that the low-viscosity RBCs have a higher proportion of organic resinous material to be activated [25] than in the high-viscosity RBC and, maybe, the light exposure time would need to be prolonged.
Although the present study aimed for simulations that more closely resemble clinical conditions, it is important to note that the conversion reading was performed immediately after the photoactivation of each increment for all tested LCUs and RBCs groups. So, the DC values found can be altered based on time and late polymerization and represents a limitation. Another limitation from the current study is that the used method does provide proper information of how the light irradiance is distributed across the wavelength bands; so additional studies are necessary with the integration of wavelength spectra analyses.
Conclusion
Considering the aforementioned results and discussion, it is therefore possible to conclude that:
regardless of the LCU used, bulk-fll composites did not present higher curing potential than the conventional composite used with the incremental technique;
the most important parameter to predict the curing ability of bulk-fll composites in deep cavities was the irradiance ratio between 6 and 0 mm depths and not solely the tip irradiance; and
that the material’s viscosity did not afect the curing potential, determined by the bottom-to-top conversion ratio.
You have the opportunity to gather more in-depth information about composite restorations in our course "International schools working with composite restorations".
List of authors:
Rodrigo Antonio Modena, Mário Alexandre Coelho Sinhoreti, William Palin, Larissa Maria Cavalcante, Luis Felipe Schneider
References
Tardem C, Albuquerque EG, Lopes LS, Marins SS, Calazans FS, Poubel LA, Barcelos R, Barceleiro MO. Clinical time and postoperative sensitivity after use of bulk-fll (syringe and capsule) vs. incremental flling composites: a randomized clinical trial. Braz Oral Res. 2019;16:e089.
Palin WM, Leprince JG, Hadis MA. Shining a light on high volume photocurable materials. Dent Mater. 2018;34:695–710.
Van Ende N, De Munck J, Lise DP, Van Meerbeek B. Bulk-fll composites: a review of the current literature. J Adhes Dent. 2017;19:95–109.
Fronza BM, Rueggeberg FA, Braga RR, Mogilevych B, Soares LE, Martin AA, Ambrosano G, Gianini M. Monomer conversion, microhardness, internal marginal adaptation, and shrinkage stress of bulk-fill resin composites. Dent Mater. 2015;31:1542–51.
Al Sunbul H, Silikas N, Watts DC. Polymerization shrinkage kinetics and shrinkage-stress in dental resin-composites. Dent Mater. 2016;32:998–1006.
Alshali RZ, Salim NA, Sung R, Satterthwaite JD, Silikas N. Qualitative and quantitative characterization of monomers of uncured bulk-fll and conventional resin-composites using liquid chromatography/mass spectrometry. Dent Mater. 2015;31:711–20.
Reis AF, Vestphal M, Amaral RCD, Rodrigues JA, Roulet JF, Roscoe MG. Efciency of polymerization of bulk-fll composite resins: a systematic review. Braz Oral Res. 2017;31(Suppl 1):e59.
Shimokawa C, Turbino ML, Giannini M, Braga RR, Price RB. Efect of curing light and exposure time on the polymerization of bulk-fll resin-based composites in molar teeth. Oper Dent. 2020;45:E141–55.
Rueggeberg FA, Giannini M, Arrais CAG, Price RBT. Light curing in dentistry and clinical implications: a literature review. Braz Oral Res. 2017;31(Suppl):e61.
Shimokawa CAK, Turbino ML, Giannini M, Braga RR, Price RB. Efect of light curing units on the polymerization of bulk fll resin-based composites. Dent Mater. 2018;34:1211–21.
Alrahlah A, Silikas N, Watts DC. Post-cure depth of cure of bulk fll dental resin-composites. Dent Mater. 2014;30:149–54.
Garcia D, Yaman P, Dennison J, Neiva GF. Polymerization shrinkage and depth of cure of bulk-fll fowable composite resins. Oper Dent. 2014;39:441–8.
Tarle Z, Attin T, Marovic D, Andermatt L, Ristic M, Tauböck TT. Infuence of irradiation time on subsurface degree of conversion and microhardness of high-viscosity bulk-fll resin composites. Clin Oral Investig. 2015;19:831–40.
Algamaiah H, Silikas N, Watts DC. Conversion kinetics of rapid photo-polymerized resin composites. Dent Mater. 2020;36:1266–74.
Kaisarly D, El Gezawi M, Keßler A, Rösch P, Kunzelmann KH. Shrinkage vectors in fowable bulk-fll and conventional composites: bulk versus incremental application. Clin Oral Investig. 2020.
Reis AF, Vestphal M, Amaral RCD, Rodrigues JA, Roulet JF, Roscoe MG. Efciency of polymerization of bulk-fll composite resins: a systematic review. Braz Oral Res. 2017;31(Suppl 1):e59.
Sahadi BO, Price RB, André CB, Sebold M, Bermejo GN, PalmaDibb RG, Faraoni JJ, Soares CJ, Giannini M. Multiple-peak and single-peak dental curing lights comparison on the wear resistance of bulk-fll composites. Braz Oral Res. 2018;17:e122.
Albuquerque PP, Bertolo ML, Cavalcante LM, Pfeifer C, Schneider LF. Degree of conversion, depth of cure, and color stability of experimental dental composite formulated with camphorquinone and phenanthrenequinone photoinitiators. J Esthet Restor Dent. 2015;27:S49-57.
Tabatabaei MH, Nahavandi AM, Khorshidi S, Hashemikamangar SS. Fluorescence and opalescence of two dental composite resins. Eur J Dent. 2019;13:527–34.
Lima RBW, Troconis CCM, Moreno MBP, Murillo-Gómez F, De Goes MF. Depth of cure of bulk fll resin composites: A systematic review. J Esthet Restor Dent. 2018;30:492–501.
Ilie N, Hickel R. Investigations on a methacrylate-based fowable composite based on the SDRTM technology. Dent Mater. 2011;27:348–55.
Fonseca AS, Labruna Moreira AD, de Albuquerque PP, de Menezes LR, Pfeifer CS, Schneider LF. Effect of monomer type on the CC degree of conversion, water sorption and solubility, and color stability of model dental composites. Dent Mater. 2017;33:394–401.
Price RB, Ferracane JL, Hickel R, Sullivan B. The light-curing unit: an essential piece of dental equipment. Int Dent J. 2020;70:407–17.
Alshali RZ, Silikas N, Satterthwaite JD. Degree of conversion of bulk-fll compared to conventional resin-composites at two time intervals. Dent Mater. 2013;29:e213–7.
Leprince JG, Palin WM, Vanacker J, Sabbagh J, Devaux J, Leloup G. Physico-mechanical characteristics of commercially available bulk-fll composites. J Dent. 2014;42:993–1000.
Tauböck TT, Jäger F, Attin T. Polymerization shrinkage and shrinkage force kinetics of high- and low-viscosity dimethacrylate- and ormocer-based bulk-fll resin composites. Odontolog. 2019;107:103–10.
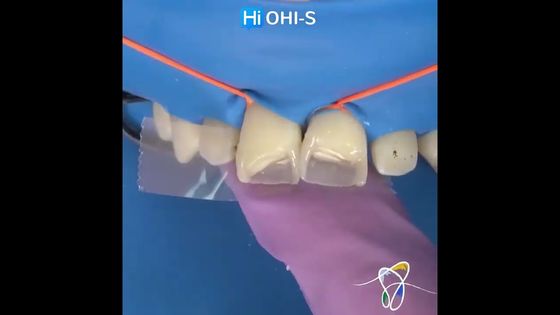
Video
OHI-S
02 April 2024
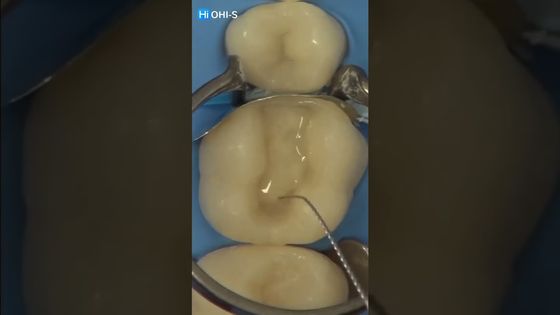
Video
OHI-S
16 January 2024
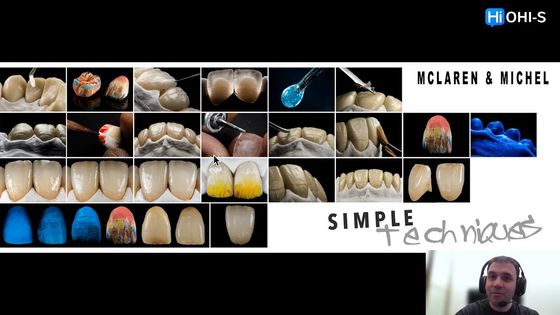
Video
OHI-S
21 November 2023