Creation of well-balanced experimental groups for comparative endodontic laboratory studies: a new proposal based on micro-CT and in silico methods
Abstract
Aim: To introduce a new method to select anatomically matched teeth using micro-computed tomographic (micro-CT) technology.
Methodology: Single-rooted mandibular incisors with a single root canal (n = 60) were selected and distributed into three experimental groups according to the method used for matching 10 pairs of teeth in each group. In group 1, the pairs of mandibular incisors were randomly selected from a pool of teeth. In group 2, teeth were paired based on the measurement of canal width 5 mm from the root apex using radiographs taken from buccolingual and mesiodistal directions. In group 3, teeth were scanned (pixel size of 14.25 lm) and pair-matched based on the anatomical aspects of the root canal, named aspect ratio (AR), volume and three-dimensional canal geometry. After allocating the specimens into groups 1 and 2, the teeth were scanned and the canal morphology evaluated as in group 3. A bivariate Pearson’s regression analysis was performed correlating the individual AR values of each pair, and the correlation coefficient was used to estimate the strength of the pair-matching process. One-way ANOVA post hoc Tukey’s tests were applied for pairwise comparisons at a significance level of 5%.
Results: The micro-CT revealed that 100% of the samples had strong (80%) or very strong (20%) correlations with respect to AR values. Analysis of the radiographic method revealed strong correlation in two pairs (20%), but most of the samples had weak (30%) or negligible (30%) correlation coefficients. The randomization method resulted in three pairs (30%) with very strong correlations, whilst 50% had weak or negligible rates. A significant difference in correlation coefficients was observed in the micro-CT method compared to the other groups (P < 0.05), whilst no difference was detected between radiographic and randomized methods (P > 0.05). Eta-squared (g2) calculations demonstrated a very high effect size in the micro-CT group for selecting pairs (0.99) and lower effect sizes in the radiographic (0.67) and randomized (0.66) groups.
Conclusions: Micro-CT method was able to provide better control of the confounding effect that anatomical variances in tooth morphology may have on the results in experiments with matched-pair design.
Introduction
Experimental bench tests have often been used to screen and rank the quality of a plethora of materials and techniques related to root canal shaping, irrigation, disinfection and filling procedures (Buck et al. 1999, Eldeniz & Ørstavik 2009, Çapar et al. 2014, Passalidou et al. 2018). However, results and conclusions from some of these comparative studies may not be reliable because of a general lack of anatomical matching of teeth prior to experimentation (De-Deus 2012). This lack of standardization reveals an inconsistent and poor internal validity of these studies, which is in line with the statement of Babb et al. (2009): ‘Although testing designs that use natural canal spaces have pragmatic appeals to clinicians, they have severe limitations from a materials science perspective’. In fact, the difficulty of creating a reliable baseline using extracted natural teeth is a consequence of the intricate anatomy of the root canal system, which is a decisive confounding factor.
Overall, experimental groups in comparative studies have been created by selecting randomized either single- or multi-rooted teeth with limited sample size (Topçuoğlu et al. 2016, Silva et al. 2017, Pedullà et al. 2019). In practice, this means a very poor standardization and the inability to ensure experimental comparability as it may yield experimental groups with large variations in the baseline of the substrate (Smith & Steiman 1994, També et al. 2014). Consequently, these studies may have been conducted under dissimilar experimental conditions and the results may reveal the effect of the root canal anatomy rather than the variable of interest, that is materials, techniques and/or instruments under comparison (De-Deus 2012). To overcome this problem and optimize the experimental design, anatomical matching of root canal morphology should be regarded as an underlying experimental first step of any comparative ex vivo study in endodontics. This will provide a consistent baseline and an improvement of overall internal validity of the study. Therefore, several studies attempted to overcome the anatomical factor using the visual examination of radiographs taken in different angulations (Yared & Bou Dagher 1994, Bürklein & Schäfer 2012) by allocating the teeth into experimental groups according to the root canal width measured at some distance from the root apex (Ruckman et al. 2013). However, the overall quality of this methodological approach is not evidence-based. One is led to speculate that this is indeed far from ideal, considering the well-known variations of canal shape throughout the root (Versiani et al. 2012, 2016a). Another approach suggests the use of contralateral teeth (Johnsen et al. 2017), which have been shown to comprise similar anatomy (Zehnder et al. 2006, Mitchell et al. 2011, Iriboz et al. 2015, Viapiana et al. 2016, Guimaraes et al. 2017). Through the use of micro-computed tomographic (micro-CT) imaging technology, it was demonstrated that contralateral premolars do indeed exhibit a high degree of matching symmetry and a valid and reliable computer simulation (in silico) method was developed for matching contralateral premolars in experimental endodontic comparative studies (Johnsen et al. 2016, 2017, 2018). These findings, however, did not preclude the use of other tooth types, but suggested that it would be conceivable to match teeth from different individuals if they are within a certain range of morphological similarity (Johnsen et al. 2016). The range, or lowest acceptable similarity coefficient, would certainly rely on further validation, and has yet to be determined. Thus, this type of database repository of non-contralateral pulp spaces would open up for extremely selective, as well as, time and cost-efficient studies.
The purpose of the present study was to introduce a novel methodology for matching non-contralateral mandibular incisors into experimental groups based on their internal morphology through the use of micro-CT imaging technology. This proposal aims to improve the internal validity of comparative studies in endodontics by the creation of anatomically well-balanced experimental groups when compared to conventional methods based on randomization or radiographic examination. To confirm the anatomical similarity of the teeth, data obtained were scrutinized with particular emphasis on the aspect ratio (AR) of the canal along the entire root length. This parameter can be considered as a token of morphological similarity between different teeth. Additionally, advantages and limitations of this new proposal were also carefully addressed. The null hypothesis tested was that there would be no difference in the correlation coefficients amongst the three tested methods.
Materials and methods
Sample size calculation
Based on the results of Versiani et al. (2013a), it has been estimated an effect size of 0.7 for the selection method to yield adequately anatomically paired samples using micro-CT technology. This value was input into a t-test family, correlation bi-serial method at G*Power for Mac 3.1 (Heinrich-Heine-Universität Düsseldorf, Düsseldorf, Germany), together with an alpha-type error of 0.5 and beta power of 95%. The software indicated a number of 16 samples (eight pairs) per group to observe significant effect of selection criteria using micro-CT over conventional methods based on randomization or radiographic examination.
Sample selection and groups
This study was approved by the local institutional ethics committee (protocol 87450517.5.0000.5243). A total of 1708 single-rooted mandibular incisors that had been stored for the last 4 years in a tooth bank of the Department of Endodontics, Fluminense Federal University, Niterói, Rio de Janeiro, Brazil, were available for this experiment. Inclusion criteria comprised only teeth showing no root fracture, calcification, caries, resorption or incomplete root formation. From this set of teeth, three experimental groups were created according to the following methods.
Group 1 – Randomized method (n = 20)
From a pool of the first 76 mandibular incisors randomly gathered from the original sample (n = 1708), it was possible to collect 20 teeth having 20 ± 1 mm in length (from the incisal edge to the anatomic apex) and randomly distributed them into two subgroups (n = 10) with the aid of a computer algorithm (http://www.random.org).
Group 2 – Radiographic method (n = 20)
From additional 100 mandibular incisors randomly gathered from the original sample (n = 1708), it was possible to select 10 pairs of teeth having similar canal width measured 5 mm from the anatomic apex (FIJI/ImageJ software v.1.51n; Fiji, Madison, WI, USA) by using digital radiographic projections (Schick CDR digital radiographic system; Dentsply Sirona, Charlotte, NC, USA) taken from both buccolingual and mesiodistal directions of each specimen.
Group 3 – Micro-CT method (n = 20)
Two-hundred and fifty-one mandibular incisors (n = 251) randomly gathered from the original sample (n = 1708) were necessary for creating of two anatomically pair-matched groups (n = 10) based on a micro-CT approach. Thus, 251 specimens were scanned (SkyScan 1173; Bruker microCT, Kontich, Belgium; 70 kV, 114 mA, pixel size of 14.25 lm, 360° rotation with a rotation step of 0.5°, frame average of five, using a 1.0-mm-thick aluminium filter) and reconstructed into axial cross-sections (NRecon v.1.7.16 software; Bruker microCT) with individualized parameters for ring artefact correction (3–4), contrast limits (0–0.05) and beam hardening correction (30–45%), resulting in 700–900 greyscale cross-section images per tooth from the cemento-enamel junction to the apex. Then, cross-sectional images were segmented to identify the root canal configuration (Figure 1a) using an automatic routine in FIJI/ImageJ software (Fiji v.1.51n; Fiji). Briefly, non-local means filter (Buades et al. 2011) was used to reduce noise whilst preserving object edges (Figure 1b), followed by applying the Otsu-based algorithm (Otsu 1979) for binarization (Figure 1c). In order to segment the root canal, the three stages of 2D ‘fill holes’ were added to the automatic routine after suitable reslicing of the volume to reveal the three orthogonal planes (xy, xz and yz). After that, small residual pixels were automatically removed with the ‘Keep Largest Region’ tool implemented in the MorphoLibJ plugin (Legland & Arganda-Carreras 2014), which allows the identification of the largest connected component, removing the disconnected ones (Figure 1d). From this point, only specimens with a single root canal (Vertucci’s type I canal configuration) were used. The volume of interest (VOI) was then established from the cemento-enamel junction to the apex for measuring the AR and volume parameters of root canal. The AR is defined as the ratio of the major to the minor diameter and was calculated at each cross-section from the apposition of an ellipse that best fits the root canal using the Shape Descriptors plugin of FIJI/ImageJ software (Fiji v.1.51n; Fiji; Figure 1e). The results of AR acquired in all slices were plotted into a graph (Figure 1f) to describe the variations of 2D geometry of the canal throughout the root. Canals with AR close to 1 present a rounded shape, whilst AR values higher than three indicate oval or elongated canal form. Volume (in mm3) was calculated as the volume of the binarized canal within the VOI using the Objects Counter tool (FIJI/ImageJ software). Three-dimensional (3D) models of the root and root canal of each specimen were also created using the CTAn v.1.18.8 software (Bruker microCT) and qualitatively evaluated from both buccal and proximal views with CTVol v.3.3 software (Bruker microCT; Figure 1g). After that, the allocation of samples into anatomical pairs was performed. First, subgroups of teeth were created according to the root canal volume with a maximum variation range of 2 mm3. It was determined with the aid of a proper statistical test (Cronbach’s alpha test) that indicated a very high homogeneity of the data (0.968) when samples were categorized within this maximum volume variation. Further, the 2D geometry of the entire root canal, represented by the AR graphical curve (Figure 1f), was compared. Teeth categorized within the same canal volume range and showing similar graphical curves were re-grouped. Finally, the 3D morphological aspect of the root canals (Figure 1g) in these groups was examined and the specimens allocated into two groups of anatomical pair-matched teeth (n = 20) based on similar volume, AR plots and 3D renderings of the root canals. Two experienced operators independently double-checked these parameters before sample distribution.
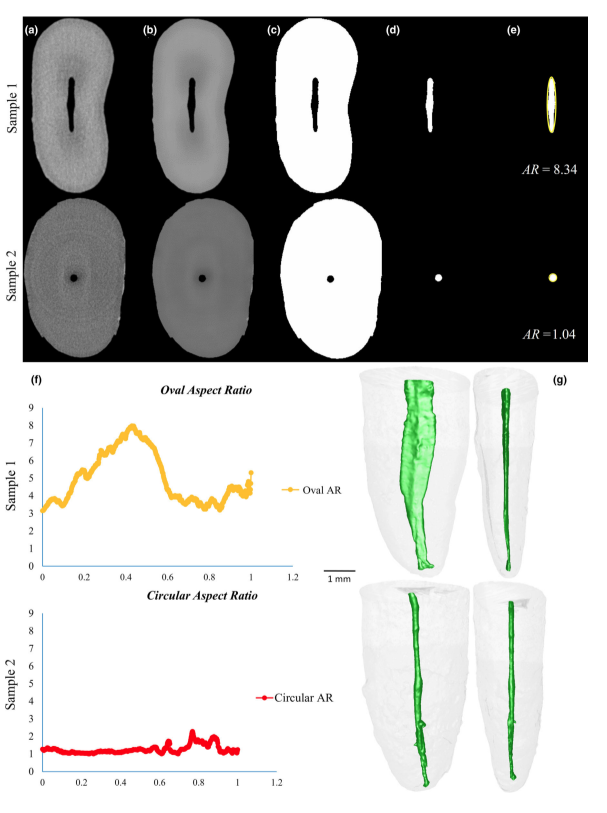
After allocating all of the specimens into the three experimental groups, the teeth selected in groups 1 and 2 were also scanned and reconstructed with the SkyScan 1173 micro-CT device (Bruker microCT) following the same parameters used in group 3. Then, AR values in each cross-section, as well as, the volume and 3D configuration of the root canals were obtained from the specimens of groups 1 and 2 and used for comparison. A detailed flow chart of the methodology is shown in Figure 2.
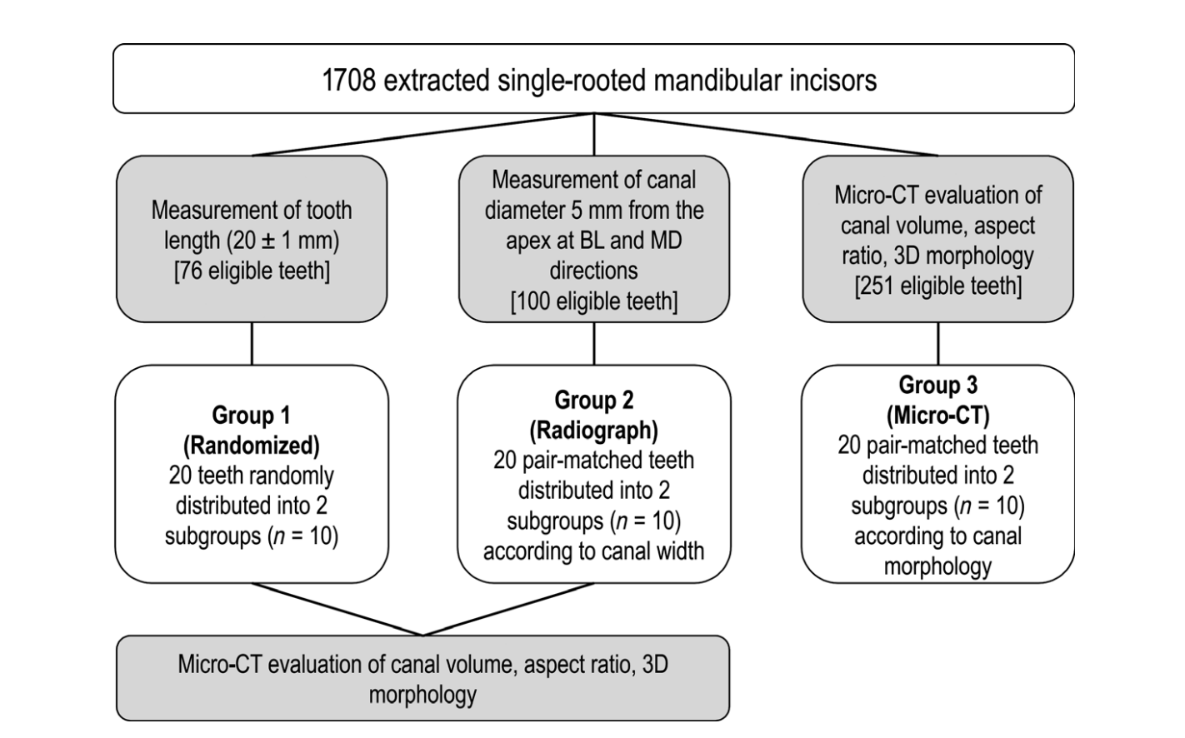
Statistical analysis
A bivariate Pearson’s regression analysis was performed correlating the individual AR values of each pair. The correlation coefficient obtained for each pair was used to estimate the strength of the pair matching alongside the root lengths following the general rule of correlations strength and categorized as very strong (0.9–1.0), strong (0.7–0.9), moderate (0.5–0.7), weak (0.3–0.5) or negligible (0–0.3; Cohen 1988). Then, the correlation coefficients were compared amongst the groups to verify the similarity of their strength regarding the methods used to form pair-matched samples. Because a bell-shaped distribution was observed for the correlation coefficients, a one-way ANOVA procedure was performed followed by a Tukey’s HSD test for pairwise comparisons. Additionally, the effect size of each method was calculated using the eta squared (g2). All statistical analyses were performed using the Statistical Package for Social Sciences software (SPSS v.24; SPSS Inc., Chicago, IL, USA) with a cut-off level for significance adopted at 5%.
Results
Table 1 shows the correlation coefficients of each pair-matched tooth using the three methods of sampling. The micro-CT method (group 3) showed 100% of the samples rating as strong (80%) or very strong (20%) correlations regarding AR values. Analysis of the radiographic method (group 2) revealed strong correlation in two pairs (20%), but most of the samples had weak (30%) or neglectable (30%) correlation coefficients. Using the randomization method (group 1), only one pair (10%) was rated at very strong correlation and two pairs (20%) at strong correlation, whilst 50% reached weak or neglectable rates. One-way ANOVA post hoc Tukey’s HSD tests found a significant difference in correlation coefficients reached by the micro-CT method compared to the other groups (P = 0.000), whilst no difference was detected between the correlations’ coefficients of the radiographic and randomized pairs (P > 0.05). Eta-squared (g2) calculations demonstrated a very high effect size in the micro-CT group for selecting pairs (0.99) and lower effect sizes in the radiographic (0.67) and randomized (0.66) methods. Figures 3, 4 and 5 illustrate the results obtained from representative pair-matched samples in each group.
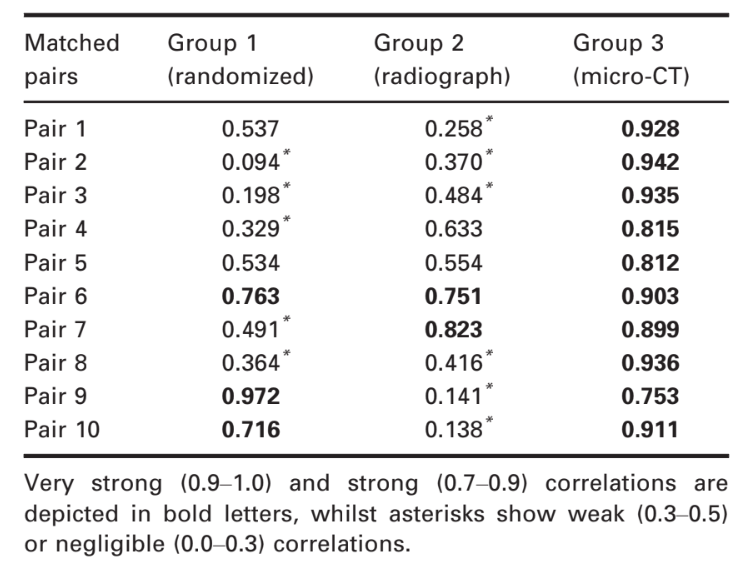
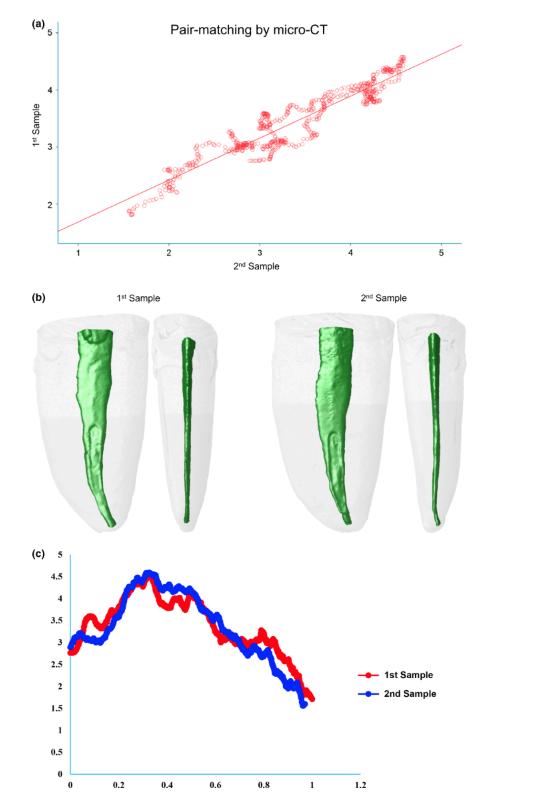
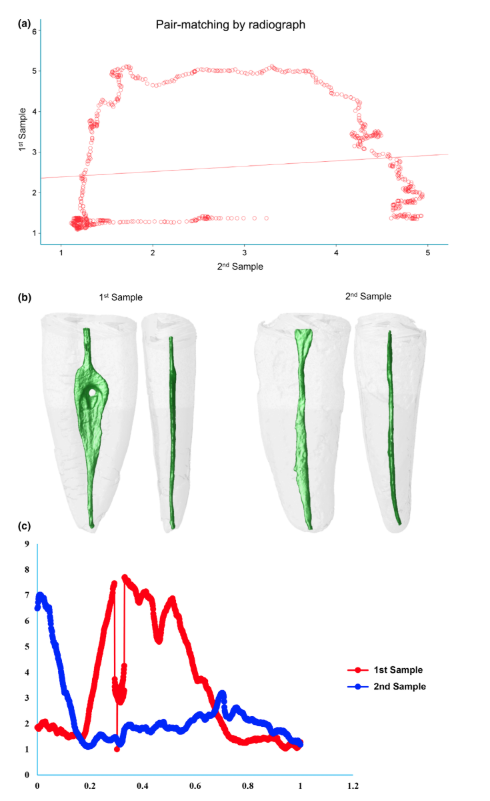
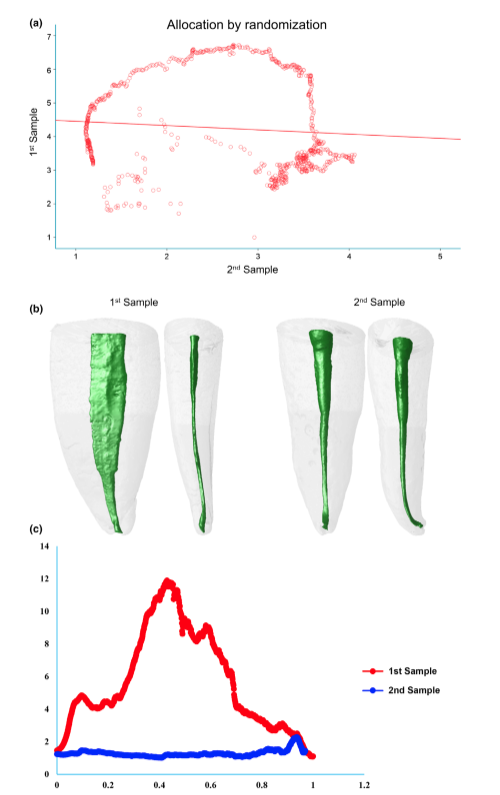
Discussion
The major challenge in creating well-balanced experimental groups for comparative endodontic studies is the variations in the intricate anatomy that may exist in a randomized group of teeth. Therefore, one important goal of ex vivo laboratory experiments must be to create a feasible method able to overcome the inherent internal variation in natural human teeth (Versiani et al. 2013a). Prior to the development of the present proposal, a literature search was conducted to identify the most commonly methods used to create pair-matched teeth samples in experimental studies in endodontology. Basically, these methods aimed to allocate samples into experimental groups according to their anatomical characteristics. It was observed that some studies adopted one point of the root, usually 5 mm from the anatomical apex, and measure the width of the root canal in both buccal and proximal directions, following the methodology of Wu et al. (2000), to determine its shape (Tinoco et al. 2014, Teixeira et al. 2015, Lee et al. 2019). In other studies, specimens are allocated from the same group of teeth by randomization (Topçuoğlu et al. 2016, Silva et al. 2017, Pedullà et al. 2019), whilst some of them adopted a combination of radiographic and randomized methods to form pairs (Ruckman et al. 2013, Bernardes et al. 2016). Recently, a few studies started to use specific anatomical parameters identified by means of a micro-CT scanning to pair-matched samples (Versiani et al. 2013b, Johnsen et al. 2016, Versiani et al. 2016b, Johnsen et al. 2017, 2018). In fact, the call for the exploration of a suitable scientific screening and matching protocol for use in endodontics comparative studies was recently raised by Xu et al. (2016) in their timely and appropriate proposal to use contralateral premolars for providing baseline consistency. Contrariwise to Johnsen et al. (2017), they found relatively few pairs of contralateral premolars with anatomical symmetry. Nevertheless, in concurrence with Johnsen et al. (2017), they found that contralateral teeth did have better symmetry than non-paired teeth. Future research should explore whether the similarity of screened and matched non-paired teeth through the present proposal of using micro-CT for pair matching is comparable with contralateral premolars. However, the availability of contralateral premolar teeth with mature apices extracted from younger patients undergoing orthodontic treatment may be limited. The present study was based on a large human ex vivo material of 1708 mandibular incisors. The teeth underwent a strict selection process to create two anatomically pair-matched groups based on micro-CT and radiographic methods and one group allocated by randomization, with each group consisting of 10 pairs of mandibular incisors. The strict selection method from a large repository of teeth along with proper a priori sample size calculation allowed for the possibility to demonstrate which group had the best well-balanced baseline experimental pairs by statistically assessing how strong is the similarity between the internal root canal shape between the pairs, based on the cross-sectional AR values correlations.
The low correlation coefficients obtained from both radiographic and randomization methods in the present study demonstrate that randomization and radiographic matching methods were unable to overcome the inherent biological variance in root canal anatomy. Thus, the null hypothesis was rejected. These results clearly demonstrated how endodontic comparative studies using unsophisticated screening and matching require an increased sample size to show real and statistically significant differences. Actually, an increase in sample size will likely lead to more precision as individual differences will matter less, but it may reach a point where the effect over precision is meaningless (Souza 2014). It must be pointed out that ethical and economic considerations are also important incentives for not having sample sizes larger than necessary. Therefore, matched and well-balanced groups can provide smaller sample sizes with sufficient power to give reliable results. In fact, the effect that baseline sample matching has on the reduction of sample size has been previously demonstrated with remarkable results in bone research (Banse et al. 1996, Barker et al. 2005).
The present methodology opens up for the future use of 3D matching and object retrieval methods (Hilaga et al. 2001, Osada et al. 2001, Tangelder & Veltkamp 2008) with deep learning or artificial neural network capabilities (Hilaga et al. 2001, Ekert et al. 2019, Krois et al. 2019). Such capabilities incorporated into a user-friendly and semi-automated interface would allow for rapid in silico selection of teeth with desired root canal morphology, such as oval-shaped canals, and then collect the samples physically from a biobank of teeth available for a multitude of different endodontic comparative experiments with high internal validity. The novel micro-CT method presented herein efficaciously removes the confounding effect that anatomical variances in root canal morphology may have on the results in pair-matched experimental designs. This will have unequivocal implications for sample distribution in experimental groups in order to improve the design of comparative studies in endodontics.
Conclusion
Use of Micro-CT was able to provide better control of the confounding effect that anatomical variances in tooth morphology may have on the results in experiments with pair-matched design.
Authors: G. De-Deus, M. Simões-Carvalho, F. G. Belladonna, M. A. Versiani, E. J. N. L. Silva, D. M. Cavalcante, E. M. Souza, G. F. Johnsen, H. J. Haugen & S. Paciornik
References:
- Babb BR, Loushine RJ, Bryan TE et al. (2009) Bonding of self-adhesive (self-etching) root canal sealers to radicular dentin. Journal of Endodontics 35, 578–82.
- Banse X, Delloye C, Cornu O, Bourgois R (1996) Comparative left-right mechanical testing of cancellous bone from normal femoral heads. Journal of Biomechanics 29, 1247– 53.
- Barker DS, Schultz C, Krishnan J, Hearn TC (2005) Bilateral symmetry of the human metacarpal: implications for sample size calculations. Clinical Biomechanics 20, 846–52.
- Bernardes RA, Duarte MAH, Vivan RR, Alcalde MP, Vasconcelos BC, Bramante CM (2016) Comparison of three retreatment techniques with ultrasonic activation in flattened canals using micro-computed tomography and scanning electron microscopy. International Endodontic Journal 49, 890–7.
- Buades A, Coll B, Morel JM (2011) Non-local means denoising. Image Processing on Line 1, 208–12.
- Buck R, Eleazer PD, Staat RH (1999) In vitro disinfection of dentinal tubules by various endodontics irrigants. Journal of Endodontics 25, 786–8.
- Bürklein S, Schäfer E (2012) Apically extruded debris with reciprocating single-file and full-sequence rotary instrumentation systems. Journal of Endodontics 38, 850–2.
- Çapar ID, Ertas H, Ok E, Arslan H, Ertas ET (2014) Comparative study of different novel nickel-titanium rotary systems for root canal preparation in severely curved root canals. Journal of Endodontics 40, 852–6.
- Cohen J (1988) Statistical Power Analysis for the Behavioral Sciences, 2nd edn. Hillsdale, NJ: L. Erlbaum Associates.
- De-Deus G (2012) Research that matters – root canal filling and leakage studies. International Endodontic Journal 45, 1063–4.
- Ekert T, Krois J, Meinhold L et al. (2019) Deep learning for the radiographic detection of apical lesions. Journal of Endodontics 45, 917–22 e5.
- Eldeniz AU, Ørstavik D (2009) A laboratory assessment of coronal bacterial leakage in root canals filled with new and conventional sealers. International Endodontic Journal 42, 303–12.
- Guimaraes LS, Gomes CC, Marceliano-Alves MF, Cunha RS, Provenzano JC, Siqueira JF Jr (2017) Preparation of oval-shaped canals with TRUShape and Reciproc systems: a micro-computed tomography study using contralateral premolars. Journal of Endodontics 43, 1018–22.
- Hilaga M, Shinagawa Y, Kohmura T, Kunii TL (2001) Topology matching for fully automatic similarity estimation of 3d shapes. In: Proceedings of the 28th Annual Conference on Computer Graphics and Interactive Techniques (SIGGRAPH ’01). New York: ACM, pp. 203–12.
- Iriboz E, Bayraktar K, Turkaydin D, Tarcin B (2015) Comparison of apical extrusion of sodium hypochlorite using 4 different root canal irrigation techniques. Journal of Endodontics 41, 380–4.
- Johnsen GF, Sundnes J, Wengenroth J, Haugen HJ (2016) Methodology for morphometric analysis of modern human contralateral premolars. Journal of Computer Assisted Tomography 40, 617–25.
- Johnsen GF, Dara S, Asjad S, Sunde PT, Haugen HJ (2017) Anatomic comparison of contralateral premolars. Journal of Endodontics 43, 956–63.
- Johnsen GF, Sunde PT, Haugen HJ (2018) Validation of contralateral premolars as the substrate for endodontic comparison studies. International Endodontic Journal 51, 942– 51.
- Krois J, Ekert T, Meinhold L et al. (2019) Deep learning for the radiographic detection of periodontal bone loss. Scientific Reports 9, 8495.
- Lee OYS, Khan K, Li KY et al. (2019) Influence of apical preparation size and irrigation technique on root canal debridement: a histological analysis of round and oval root canals. International Endodontic Journal 52, 1366–76.
- Legland D, Arganda-Carreras I. (2014) MorphoLibJ. [WWW document]. https://imagej.net/MorphoLibJ#Application_ to_binary_images [accessed on September 1st 2019].
- Mitchell RP, Baumgartner JC, Sedgley CM (2011) Apical extrusion of sodium hypochlorite using different root canal irrigation systems. Journal of Endodontics 37, 1677–81.
- Osada R, Funkhouser T, Chazelle B, Dobkin D (2001) Matching 3d models with shape distributions. In: Proceedings International Conference on Shape Modeling and Applications, vol.1, pp. 154–68.
- Otsu N (1979) A threshold selection method from grey level histograms. IEEE Transactions on Systems, Man, and Cybernetics 9, 62–6.
- Passalidou S, Calberson F, De Bruyne M, De Moor R, Meire MA (2018) Debris removal from the mesial root canal system of mandibular molars with laser-activated irrigation. Journal of Endodontics 44, 1697–701.
- Pedullà E, Abiad RS, Conte G et al. (2019) Retreatability of two hydraulic calcium silicate-based root canal sealers using rotary instrumentation with supplementary irrigant agitation protocols: a laboratory-based micro-computed tomographic analysis. International Endodontic Journal 52, 1377–87.
- Ruckman JE, Whitten B, Sedgley CM, Svec T (2013) Comparison of the self-adjusting file with rotary and hand instrumentation in long-oval-shaped root canals. Journal of Endodontics 39, 92–5.
- Silva EJ, Perez R, Valentim RM et al. (2017) Dissolution, dislocation and dimensional changes of endodontic sealers after a solubility challenge: a micro-CT approach. International Endodontic Journal 50, 407–14.
- Smith MA, Steiman HR (1994) An in vitro evaluation of microleakage of two new and two old root canal sealers. Journal of Endodontics 20, 18–21.
- Souza E (2014) Research that matters: setting guidelines for the use and reporting of statistics. International Endodontic Journal 47, 115–9.
- També VH, Nagmode PS, Abraham S, Patait M, Lahoti PV, Jaju N (2014) Comparison of canal transportation and centering ability of rotary protaper, one shape system and wave one system using cone beam computed tomography: an in vitro study. Journal of Conservative Dentistry 17, 561–5.
- Tangelder JH, Veltkamp RC (2008) A survey of content based 3d shape retrieval methods. Multimedia Tools and Applications 39, 441–71.
- Teixeira JM, Cunha FM, Jesus RO, Silva EJ, Fidel SR, Sassone LM (2015) Influence of working length and apical preparation size on apical bacterial extrusion during reciprocating instrumentation. International Endodontic Journal 48, 648–53.
- Tinoco JM, De-Deus G, Tinoco EM, Saavedra F, Fidel RA, Sassone LM (2014) Apical extrusion of bacteria when using reciprocating single-file and rotary multifile instrumentation systems. International Endodontic Journal 47, 560–6.
- Topçuoğlu HS, Zan R, Akpek F et al. (2016) Apically extruded debris during root canal preparation using Vortex Blue, K3XF, ProTaper Next and Reciproc instruments. International Endodontic Journal 49, 1183–7.
- Versiani MA, Pécora JD, Sousa-Neto MD (2012) Root and root canal morphology of four-rooted maxillary second molars: a micro-computed tomography study. Journal of Endodontics 38, 977–82.
- Versiani MA, Pécora JD, Sousa-Neto MD (2013a) Microcomputed tomography analysis of the root canal morphology of single-rooted mandibular canines. International Endodontic Journal 46, 800–7.
- Versiani MA, Steier L, De-Deus G, Tassani S, Pécora JD, Sousa-Neto MD (2013b) Micro-computed tomography study of oval-shaped canals prepared with the Self-adjusting File, Reciproc, WaveOne, and Protaper Universal systems. Journal of Endodontics 39, 1060–6.
- Versiani MA, Ahmed HM, Sousa-Neto MD, De-Deus G, Dummer PM (2016a) Unusual deviation of the main foramen from the root apex. Brazilian Dental Journal 27, 589–91.
- Versiani MA, Alves FR, Andrade-Junior CV et al. (2016b) Micro-CT evaluation of the efficacy of hard-tissue removal from the root canal and isthmus area by positive and negative pressure irrigation systems. International Endodontic Journal 49, 1079–87.
- Viapiana R, Moinzadeh AT, Camilleri L et al. (2016) Porosity and sealing ability of root fillings with gutta-percha and BioRoot RCS or AH Plus sealers. Evaluation by three ex vivo methods. International Endodontic Journal 49, 774– 82.
- Wu MK, R’Oris A, Barkis D, Wesselink PR (2000) Prevalence and extent of long oval canals in the apical third. Oral Surgery Oral Medicine Oral Pathology Oral Radiology and Endodontics 89, 739–43.
- Xu J, Shao MY, Pan HY et al. (2016) A proposal for using contralateral teeth to provide well-balanced experimental groups for endodontic studies. International Endodontic Journal 49, 1001–8.
- Yared GM, Bou Dagher FE (1994) Apical enlargement: influence on the sealing ability of the vertical compaction technique. Journal of Endodontics 20, 313–4.
- Zehnder M, Luder HU, Schatzle M, Kerosuo E, Waltimo T (2006) A comparative study on the disinfection potentials of bioactive glass S53P4 and calcium hydroxide in contra-lateral human premolars ex vivo. International Endodontic Journal 39, 952–8.