Influence of Resin Matrix on the Rheology, Translucency, and Curing Potential of Experimental Flowable Composites for Bulk-Fill Applications
Abstract
Objective. To propose monomer formulations that show an optimal degree of conversion as a function of depth for bulk-fill applications. And more details about composite materials and protocols of direct restorations are accessible for you to learn in our course "International schools working with composite restorations".
Methods. Four resin blends were formulated with methacrylate-based monomers: BisGMA + TEGDMA (control); BisEMA + BisGMA + TEGDMA (BisEMA-based); UDMA + BisGMA + TEGDMA (UDMA-based) and BisEMA + UDMA + BisGMA + TEGDMA (BisEMA + UDMA-based). For each material, a photoinitiating system and silanized filler particles were added. The rheological analyses were performed with a rotational rheometer using the cone/plate geometry. CIELab coordinates were assessed over black and white backgrounds using a bench spectrophotometer (SP60, X-Rite) to calculate the translucency parameter (TP) for samples with 0.5, 4, and 6 mm thickness. The degree of C C conversion (DC) was determined by infrared spectroscopy (FTIR/ATR) at 0.05 mm (top), 4, and 6 mm depths (bottom), and the bottom-to-top ratio was considered. A broad spectrum–based LED was used for light activation. Analysis of variance and Tukey’s test (95%) were performed on the results.
Results. The materials tested showed pseudoplastic and thixotropic behavior and a predominance of viscous effects over elastics. The control resin yielded the lowest viscosity for the entire shear rate investigated, followed by the BisEMA-based, BisEMA + UDMA-based, and UDMA-based group, which had the highest viscosity. The UDMA-based material showed the lowest TP as a function of thickness. Both the materials’ formulations and depths significantly influenced the DC. The UDMA-based group promoted the highest DC on the top (71 ± 1%) and 4 mm depth (68 ± 1%) but exhibited lower bottom-to-top DC ratio. The BisEMA + UDMA-based material promoted the highest bottom-to-top DC ratio at 4 mm (99%) and 6 mm (97%).
1. Introduction
Bulk fill is a recently developed approach to dental composites, and the industry has broadened its possibilities for clinical use [1,2]. Whereas conventional composites require a restorative protocol with up to 2 mm-based increments to reduce polymerization stress and improve the depth of cure, bulk-fill composites can be used in increments of 4–5 mm with acceptable properties for the final restoration [3–5]. Indeed, the greatest advantage is the significant reduction in the clinical time required to perform the reconstruction of the lost structure [6,7].
In many cases, marketing campaigns of some companies have focused on innovations in their respective formulations, in particular by the use of specific monomers or novel initiating systems [8]. However, bulk-fill composites were developed objectively by the increase in material translucency, either by the use of less opaque filler particles or by the adoption of particles that have refractive indices compatible with the resinous matrix [9,10]. In addition,the application of monomers already used in conventional resin formulations, especially urethane dimethacrylate (UDMA) or variations of these, is another common strategy of manufacturers [3,8]. Despite all developments, laboratory studies that investigate bulk-fill composites often show discrepancies in results or concepts [5]. Some inconsistencies are due to the use of commercially available resin composites, which have variations in materialformulations, making it difficultto determine the influence of a specific component. Additionally, bulk-fill composites can also differ in viscosity [2,3,11–13], which can completely change the technique of the material application.
The material’s rheological behavior can be altered by the composition of the employed monomers [14–21], and, with the differences in their reactivity and refractive indices, the ratio of monomers employed may also alter the depth of cure. Facing this scenario, the development of experimental resin formulations for bulk-fill applications is fundamental to better understand the effects of each component on the material behavior and, more importantly, the effect of innovative components in the field of such applications. Therefore, the novelty, the main objective of this work, lies in developing a formulation using monomers that are commonly employed by researchers and dental manufacturers. Specifically, the current research aims to characterize the rheological behavior, translucency parameter (TP), and curing potential of experimental resin composites formulated with variations in the resinous matrix. The research hypothesis is that combinations of monomers that have been traditionally used in manufacturing resin composites are sufficient to achieve a proper depth of cure for bulk-fill applications.
2. Materials and methods
2.1. Materials tested
Four groups of bulk resins were made using the following monomers and respective amount (in wt%):
Bisphenol A diglycidildimethacrylate (BisGMA, refractive index = 1.549) + Triethylene glycol dimethacrylate (TEGDMA, refractive index = 1.459) — (control): BisGMA and TEGDMA (70:30 wt%).
Bisphenol A diethoxydildimethacrylate BisEMA + BisGMA + TEGDMA (BisEMA-based group): BisEMA (refractive index = 1.535), BisGMA and TEGDMA (65:29:6 wt%).
Urethane dimethacrylate (UDMA)+BisGMA + TEGDMA (UDMA-based group): UDMA (refractive index = 1.483), Bis-GMA and TEGDMA (65:29:6 wt%). 4 BisEMA + UDMA + BisGMA + TEGDMA (BisEMA + UDMA-based group): BisEMA, UDMA, BisGMA, TEGDMA (32.5:32.5:29:6 wt%).
Fig. 1 details the molecular structure of the monomers used in this study. For each material, a photoinitiating system consisting of camphorquinone (CQ, 0.1 wt%); and ethyl 4-dimethylaminobenzoate (EDMAB, 0.3 wt%), and silane-treated filler particles (0.7 - m, Esstech Inc., refractive index = 1.553, 44% by mass. 23% by volume) were added.
Fig. 1 – Molecular structure of the monomers used in this study.
2.2. Rheological behavior
A rotational rheometer (Haake, MARS III, Thermo Fisher Scientific, Waltham, MA, USA) was used to determine the rheological behavior. In addition to a thermostatic bath, the instrument has an internal electrical resistance system that ensures precise temperature control. The cone-plate measuring system used consists of a cone that rotates at the top and a stationary plate mounted on the rheometer support with the sample contained between them. The drag flow is imposed by the rotation (in the case of permanent shear flow) of the cone at a shear rate or oscillation (in the case of oscillatory flow) of the cone at an angular velocity. The sensor used in rheological measurements (C35/1◦ Ti) has a diameter ø = 35 mm and a cone angle ˛ = 1◦.
The experimental samples were prepared: sample 01 (BisGMA + TEGDMA, control group), sample 02 (BisEMA-based group), sample 03 (UDMA-based group), and sample 04 (BisEMA + UDMA-based group). Stationary (flow curve) and oscillatory (stress sweep and frequency sweep) tests were performed at 25 ± 0.2 ◦C to obtain the following rheological properties: viscosity, storage module (elastic), loss modulus (viscous), complex modulus, complex viscosity, and phase angle.
2.3. Translucency parameter
Three samples (n = 3) of each resin composite were made with 0.5, 4, and 6 mm thickness (ø = 8 mm). The materials were photoactivated by one light source (Valo, Ultradent, South Jordan, UT, USA) with 1200 mW/cm2 irradiance for 20 s. CIELab coordinates—where L* indicates the sample’s lightness, and the chromaticity coordinates a* represent the red–green axis and b* the yellow–blue axis—were determined 24 h after the photoactivation procedures with a bench spectrophotometer (SP60, X-Rite, Grand Rapids, MI, USA) over black and white backgrounds (ColorChecker; X-Rite). TP was calculated according to the following formula:
2.4. Degree of C=C conversion
The degree of conversion (n = 3) was assessed by Fourier transform infrared spectroscopy (FTIR) using the attenuated total reflectance (ATR) technique, with 16 scans and 4 cm−1 resolution (Alpha; Bruker Optics, Ettlingen, Germany). The degree of conversion was determined at different depths: 0.05 mm to simulate the region near the outer surface of a restoration, 4 mm, and 6 mm to simulate the bottom of deep cavities. Readings were taken before and after the 20-s photoactivation process, generating a graph in each process, which analyzed the area of two peaks—1637 cm−1 (aliphatic bonds) and 1608 cm−1 (bonds). For the analysis of the degree of conversion to compare to the graph, Equation (1) was used:
where DC corresponds to the degree of conversion; “Abs 1637 cm— 1 polymerized” refers to the value of the peak area of absorption ofthe aliphatic bonds C C ofthe polymerized sample; “Abs 1608 cm— 11 polymerized” relates to the value of the aromatic ring absorption peak area of the polymerized samples; “Abs 1637 cm— 1 unpolymerized” matches the peak area absorption value of the aliphatic bonds C C of the unpolymerized sample; “Abs 1608 cm— 1 unpolymerized” corresponds to the value of the aromatic ring absorption peak area of the unpolymerized samples. Data were analyzed in a two-way analysis of variance (ANOVA) followed by the Tukey’s test (95% significance).
3. Results
Table 1 lists materials’ viscosity values, degrees of conversion as a function of depth, and bottom-to-top ratios. For the characterization of the rheology behavior, Fig. 2 shows the flow curves of the BisEMA-based group, UDMA-based group, BisEMA/UDMA-based group, and control, which all have nearly constant viscosities up to 500 s−1 at 25 ◦C. As the shear rate increases beyond 500 s−1, the resins exhibit pseudoplastic behavior characterized by decreasing viscosity. The control resin has the lowest viscosity for the entire shear rate investigated, followed by the BisEMA-based, BisEMA + UDMA-based, and UDMA-based groups, respectively. The average viscosities for the resins at 25 ◦C in the shear rate range of 1–500 s−1 were 1.130, 1.604, 2.610, and 4.345 Pa·s for the control, BisEMA-based; BisEMA + UDMA-based, and UDMA-based materials, respectively. Fig. 2 also shows the thixotropic behavior (decrease in viscosity over time for a given shear rate) at 25 ◦C of the four resins. The hysteresis area (the area between curves) is larger for the less viscous resins. The stress sweep tests at 25 ◦C were performed to verify the linear viscoelastic region, corresponding to the constant values of the viscous and elastic moduli G” and G’, and to identify the stress values for the frequency sweep tests; in this case, 5 Pa was chosen, for which the values of G” and G’ are constant, as shown in Fig. 3.
Fig. 4 shows the results of resin frequency sweep tests. The following rheological properties were obtained: complex viscosity*; viscous modulus G” and elastic modulus G’. For all resins in the angular velocity range investigated, the viscous effects were more prevalent than the elastic effects (G” > G’), and elastic effects become more significant for angular velocity values greater than 10 rad/s. The complex viscosity remained nearly constant during the test, showing a slight decrease for angular velocity values above 300 rad/s.
Fig. 5 shows the phase angles obtained in the frequency sweep test at 25 ◦C and 5 Pa. Phase angles less than 90◦ exhibited a viscoelastic behavior of the material. In the case of the resins in question, the viscoelastic behavior was noted for angular velocity values greater than 10 rad/s, during which the control resin had the lowest phase angles, followed by BisEMA-based, BisEMA + UDMA-based, and UDMA-based groups, respectively.
Fig. 6 shows the viscosity obtained from the flow curve test at 25 ◦C and the complex viscosity determined in the frequency sweep test. Resins tend to follow the Cox-Merz rule, which states that equal values of viscosity and complex viscosity are evaluated at the same values of shear rate (1/s) and angular velocity (rad/s).
Fig. 7 details the TP as a function ofthickness for each materialtested. The control group yielded the highest TP, regardless ofthickness. On the opposite side,the UDMA-based group presented the lowest values for samples at 4 and 6 mm depth. Furthermore, this group showed the greatest increase in opacity as a function of thickness, with approximately one-third the TP compared to that of the subsurface (0.05) at 4 mm thickness and 5% at 6 mm.
Both the formulation of the resin matrix and the thickness analyzed significantly influenced the degree of conversion (p < 0.05), as well as the interaction (p = 0.016) (Table 1). The material formulated with the addition of UDMA promoted the conversion values statistically higher than the others on the top and 4 mm depth, while the BisEMA + UDMA-based material promoted the highest values at 6 mm depth. The highest polymerization potential as a function of depth was obtained with the BisEMA + UDMA-based material (99% at 4 mm and 97% at 6 mm).
Table 1 – Material viscosities, degree of conversion as a function of depth, and bottom-to-top ratios for 4 and 6 mm depths.
Fig. 2 – Resins flow curve and thixotropy—BisEMA, UDMA, BisEMA + UDMA-based materials, and control at 25 ◦C.
Fig. 3 – Resins stress sweep—(A) control, (B) BisEMA, (C) BisEMA + UDMA, and (D) UDMA-based materials at 25 ◦C and 1 Hz.
Fig. 4 – Frequency sweep of resins—(A) control, (B) BisEMA, (C) BisEMA + UDMA, and (D) Uma-based materials at 25 ◦C and 5 Pa.
Fig. 5 – Phase angle of resins—BisEMA, UDMA, BisEMA + UDMA-based groups, and control at 25 ◦C and 5 Pa.
Fig. 6 – Cox-Merz rule of (A) control, (B) BisEMA, (C) BisEMA + UDMA, and (D) UDMA-based groups at 25 ◦C.
Fig. 7 – Translucency parameter as a function of material and thickness evaluated.
4. Discussion
Rheology is fundamental in the development of new products, the optimization of processes, and the characterization of materials, as it studies the behavior of materials subjected to flow and deformation. Through the rheological tests and the determination of the various rheological properties of the materials subjected to different flow conditions, relevant information is obtained for different applications of scientific and technological interests, such as those related to the dental materials [20,21]. In the present work, the rheology of experimental bulk-fill composites was characterized. Thus, for each material, the flow curve, stress sweep, and frequency sweep were assessed to characterize the viscosity, elastic modulus, viscous modulus, and complex viscosity and phase angle. The control resin had the lowest viscosity for the entire shear rate investigated. The BisGMA monomer, introduced into Bowen’s resin composite matrix in 1963, has a high molecular weight and increases material viscosity, owing to its strong intramolecular hydrogen bonding. Therefore, diluent monomers such as TEGDMA are usually added because of their molecular flexibility [19]. The addition of TEGDMA allows the reduction of the viscosity from the co-monomer blend as seen in the current investigation where the control group presents a high content of this component and the lowest viscosity [17]. The addition of BisEMA and UDMA increased the viscosity for all three groups; from least to highest viscosity, they are BisEMA-based, BisEMA + UDMA-based, and UDMA-based.
The resins showed pseudoplastic and thixotropic behavior and a predominance of viscous effects over elastics. Furthermore, the materials presented nearly constant viscosities up to 500 s−1 for a temperature of 25 ◦C. For higher shear rates, the greater alignment of the macromolecules caused less flow resistance and consequently a decrease in viscosity.
Regarding thixotropy, the hysteresis area is larger for less viscous resins. For all resins in the investigated angular velocity range, viscous effects were more prevalent than the elastic effects (G”> G’), and elastic effects became more significant for angular velocity values greater than 10 rad/s. The complex viscosity remained nearly constant during the test, showing a slight decrease for angular velocity values above 300 rad/s.
The plateau in the complex viscosity value was also reported by Lee et al. [20] in the case of the monomers blends BisGMA and TEGDMA at 25 ◦C, and Petrovic et al. [21] found similar outcomes in the case of the commercial resin Smart Dentin Replace (posterior bulk-fill flowable base) at 23 ◦C, resulting with G”> G’. The analyzed resins in the present work tend to follow the Cox-Merz rule (equal values of viscosity and complex viscosity are evaluated at the same values of shear rate (1/s) and angular velocity (rad/s)). This trend was also pointed out by Lee et al. [20] in the case of the monomers blends BisGMA and TEGDMA at 25 ◦C.
TP indicates the ability to transmit light through a given material. The current outcomes indicate that the addition of BisEMA and especially UDMA to the BisGMA + TEGDMA mixture reduced the TP, i.e., increased opacity. This behavior is probably associated with the refractive index of each monomer and its relationship with the refractive index of the filler particle employed. Thus, the greater the difference, the greater the light scattering and the lower the penetration depth of light. Therefore, as UDMA is the monomer with the highest difference in relation to the filler particle, its addition negatively impacted the passage of light, especially at the 6 mm depth. Miletic et al. [22] evaluated the refractive indices (RIs) and translucency of unfilled resin mixtures and resin-based composites and found similar outcomes. Another possibility, which requires a more precise evaluation, is that the high viscosity imposed by UDMA generated a higher amount of air bubble entrapment in the mixture and, thus, elevated the light scattering effect.
The degree of C C conversion analysis was performed to determine to what extent monomers were converted by light activation as a function of depth. Bottom-to-top conversion ratios of 99% and 97% at 4 and 6 mm depth were obtained, respectively, with the addition of BisEMA and UDMA to a standard mixture with BisGMA and TEGDMA. Therefore, the research hypothesis was validated—a proper combination of monomers that are traditionally used in resin composite manufacturing is sufficient to achieve proper depth of cure.
The UDMA-based material showed the highest degree of C C conversion when compared to the others at 0.05 and 4 mm depths and a high bottom-to-top DC ratio at a 4 mm depth. This monomer presents a flexible structure, and in the polymerization process, hydrogen atoms that are weakly bonded to nitrogen come off during this polymer chain propagation and aid in the transmission of the polymer chain, improving the conversion of the material [17–19]. Furthermore, CQ was shown to interact with UDMA and start the polymerization reaction without the presence of an amine-based co-initiator [23] an additional factor in raising the medium reactivity when UDMA is added. This result corroborates with the research by Zorzin et al. [13], who studied polymerization properties of bulk-fill composites and found thatthe resinous matrix has a directinfluence on the degree of conversion of selected composites. In their study, many composites were formulated with UDMA and BisGMA, and those materials formulated with UDMA yielded a higher polymerization capacity than those in which BisGMA dominated the resinous part. On the other hand, the UDMA-based formulation showed a large drop in the polymerization potential when analyzed at a depth of 6 mm in the current study, resulting in the lowest light transmission capacity as a function of depth.
The BisEMA-based group behaved similarly to the control group in terms ofthe degree of conversion and curing potential as a function of depth. This monomer is an analog of Bis-GMA [19,24] and has a lower viscosity compared to BisGMA and UDMA because of the low secondary molecular interactions with hydroxyl groups that it presents [17–20]. Similar to the control group, the low viscosity allows the formation of a very high, crosslinked network that restricts the molecular mobility and impairs the polymerization process, resulting in reduced material conversion when depth is increased. Therefore, the balance of the flowable monomer, BisEMA, with the very reactive but more viscous UDMA promotes the best compromise for a solution. A further advantage of the combined addition of BisEMA and UDMA over the UDMA-based group is that the lower viscosity ofthe blend allows more filler particles to be incorporated, improving the physicochemical properties of the resulting material.
The availability of experimental (or model) bulk-fill formulations is valuable for the scientific community to use as a basis for understanding, applying, and developing materials for this clinical application format. Thus, the goal of the current research is to present a basic formulation from the combination of BisGMA and TEGDMA with BisEMA and UDMA. Although the achievement, this study presents some limitations, including the fact that only one filler particle was used. Thus, new studies should be carried out with the addition of other inorganic components. Furthermore, the analyses of polymerization kinetics, shrinkage-stress, physicochemical properties, and resistance to degradation should also be considered.
5. Conclusions
The research hypothesis—appropriate combinations of monomers that are traditionally used in resin composite manufacturing are sufficient to achieve proper depths of cure for bulk-fill applications— was confirmed.
The resin matrix interferes in the rheological behavior, the translucency parameter, and polymerization capacity as a function of depth.
The BisEMA + UDMA-based material demonstrated the highest curing potential as a function of depth.
If you enjoyed reading this article and would like to explore the composite restoration protocols further, we encourage you to enroll our course "Brazilian School of Aesthetic Restorations".
List of authors:
Leonardo Duarte Eiras Pereiraa, Mário Pereira Couto Neto, Roberto Guimarães Pereirac, Luis Felipe J. Schneider
References
Maas MS, Alania Y, Natale LC, Rodrigues MC, Watts DC, Braga RR. Trends in restorative composites research: what is in the future? Braz Oral Res 2017;31(suppl 1):e55.
Van Ende A, De Munck J, Lise DP, Van Meerbeek B. Bulk-fill composites: a review of the current literature. J Adhes Dent 2017;19(2):95–109.
Chesterman J, Jowett A, Gallacher A, Nixon P. Bulk-fill resin-based composite restorative materials: a review. BDJ 2017;222:337–44.
Benetti AR, Havndrup-Pedersen C, Pedersen MK, Honoré D, Pallesen U. Bulk-fill resin composites: polymerization contraction, depth of cure, and gap formation. Oper Dent 2015;40:190–200.
Reis AF, Vestphal M, Amaral RCD, Rodrigues JA, Roulet JF, Roscoe MG. Efficiency of polymerization of bulk-fill composite resins: a systematic review. Braz Oral Res 2017;31(suppl 1):e59.
Viana-de-Pinho MG, Rego GF, Vidal ML, Alonso RCB, Schneider LFS, Cavalcante LM. Clinical time required and internal adaptation in cavities restored with bulk-fill composites. J Contemp Dent Pract 2017;18(December (12)):1107–11.
Tardem C, Albuquerque EG, Lopes LS, Marins SS, Calazans FS, Poubel LA, et al. Clinical time and postoperative sensitivity after use of bulk-fill (syringe and capsule) vs. Incremental filling composites: a randomized clinical trial. Braz Oral Res 2019;33(September):e089.
Fronza BM, Rueggeberg FA, Braga RR, Mogilevych B, Soares LE, Martin AA, et al. Monomer conversion, microhardness, internal marginal adaptation, and shrinkage stress of bulk-fill resin composites. Dent Mater 2015;31(December (12)):1542–51.
Palin WM, Leprince JG, Hadis MA. Shining a light on high volume photocurable materials. Dent Mater 2018;34(May (5)):695–710.
Par M, Spanovic N, Mohn D, Attin T, Tauböck TT, Tarle Z. Curing potential of experimental resin composites filled with bioactive glass: a comparison between Bis-EMA and UDMA based resin systems. Dent Mater 2020;36(June (6)):711–23.
Papadogiannis D, Tolidis K, Gerasimou P, Lakes R, Papadogiannis Y. Viscoelastic properties, creep behavior and degree of conversion of bulk fill composite resins. Dent Mater 2015;31(December (12)):1533–41.
Marovic D, Tauböck TT, Attin T, Panduric V, Tarle Z. Monomer conversion and shrinkage force kinetics of low-viscosity bulk-fill resin composites. Acta Odontol Scand 2015;73(August (6)):474–80.
Zorzin J, Maier E, Harre S, Fey T, Belli R, Lohbauer U, et al. Bulk-fill resin composites: polymerization properties and extended light curing. Dent Mater 2015;31:293–301.
Camargo FM, Della Bona Á, Moraes RR, Coutinho de Souza CR, Schneider LF. Influence of viscosity and amine content on C==C conversion and color stability of experimental composites. Dent Mater 2015;31(May (5)):e109–15.
Fonseca AS, Labruna Moreira AD, de Albuquerque PP, de Menezes LR, Pfeifer CS, Schneider LF. Effect of monomer type on the CC degree of conversion, water sorption and solubility, and color stability of model dental composites. Dent Mater 2017;33(April (4)):394–401.
Palagummi SV, Hong T, Wang Z, Moon CK, Chiang MYM. Resin viscosity determines the condition for a valid exposure reciprocity law in dental composites. Dent Mater 2020;36(February (2)):310–9.
Lovell LG, Stansbury JW, Syrpes DC, Bowman CN. Effects of composition and reactivity on the reaction kinetics of dimethacrylate/dimethacrylate copolymerizations. Macromolecules 1999;32:3913–21.
Anseth KS, Wang CM, Bowman CN. Reaction behavior and kinetic constants for photopolymerization of multi(meth)acrylate monomers. Polymer 1994;35:3243–50.
Andrzejewska E. Photopolymerization kinetics of multifunctional monomers. Prog Polym Sci 2001;26(4):605–65.
Lee JH, Um CM, Lee IB. Rheological properties of resin composites according to variations in monomer and filler composition. Dent Mater 2006;22:515–26.
Petrovic LM, Zorica DM, Stojanac IL, Krstonosic VS, Hadnadjev MS, Atanackovic TM. A model of the viscoelastic behavior of flowable resin composites prior to setting. Dent Mater 2013;29:929–34.
Miletic V, Jakovljevic N, Manojlovic D, Marjanovic J, Rosic AA, Dramicanin ´ MD. Refractive indices of unfilled resin mixtures and cured composites related to color and translucency of conventional and low-shrinkage composites. J Biomed Mater Res B Appl Biomater 2017;105(1):7–13.
Asmusen S, Arenas G, Cook WD, Vallo C. Photobleaching of camphorquinone during polymerization of dimethacrylate-based resins. Dent Mater 2009;25(12):1603–11.
Alshali RZ, Salim NA, Sung R, Satterthwaite JD, Silikas N. Qualitative and quantitative characterization of monomers of uncured bulk-fill and conventional resin-composites using liquid chromatography/mass spectrometry. Dent Mater 2015;31:711–20.
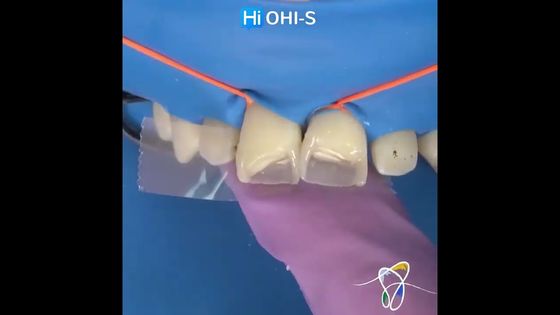
Video
OHI-S
02 April 2024
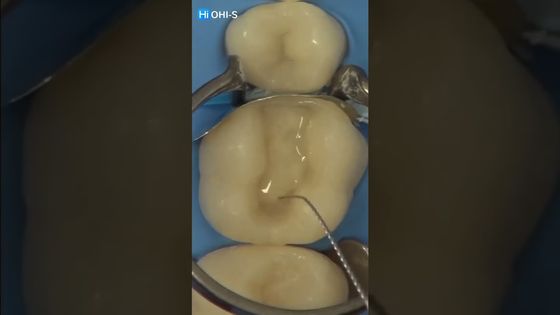
Video
OHI-S
16 January 2024
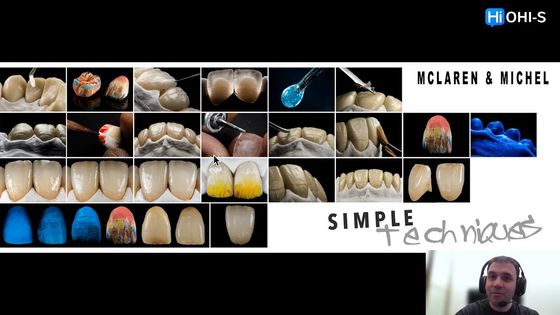
Video
OHI-S
21 November 2023