Can Bulk-Fill Compromise The Curing Potential in Deep Cavities?
ABSTRACT
Objective: To determine the effect of the restorative technique, material’s opacity and the selected light sources on the curing potential (CP) in deep cavities. Further details about composite restorations are accessible for you to learn on our website in Direct Restoration section.
Material and Methods: The radiant exitance (mW/cm2) of two light curing units (Bluephase G2 and Radii-Cal) was determined at 0 and 8 mm distance from a power meter sensor (Ophir). Two bulk-fill composites of regular consistency (Opus, FGM; and Filtek One, 3M) and a conventional one (Sirius-Z, DFL) were considered and the level of their opacity were determined by a sphere-based spectrophotometer (SP60, X-Rite). The degree of C=C conversion (DC) was determined by spectroscopy (FTIR-ATR) at 0.05 and 8 mm depths and the CP considered the ratio between them. The “incremental technique” considered 4 increments of 2mm thickness each, whereas the “bulk-fill technique considered 2 increments of 4mm-thickness. Data were submitted to analysis of variance and Tukey’s test (95%). Pearson’s correlation tests were performed to calculate the possible relation between curing potential and materials’ opacity.
Results: With the incremental technique it was possible to achieve high CP regardless of the light source. The CP of bulk-fill composites was dependent on the light source, whereas Radii-Cal compromised the DC at 8 mm for bot materials. The correlation between opacity and CP was dependent on the light source (r = 0.891707246 for Radii-Cal; r = 0.515703768 for Bluephase G2).
Conclusion: The bulk-fill technique was dependent on the light source while the incremental was not. The influence of materials’ opacity was dependent on the light curing unit.
INTRODUCTION
Resin composites have become widespread used in the dental practice due to the ability to naturalness restore anterior and posterior teeth in a single session, in a practical and functional way at an affordable price and with less operative time, when compared to ceramic materials [1,2].
Despite the evolution over the years, this material still had some disadvantages, such as polymerization stress and low activation capacity at great depths [3]. To reduce these shortcomings, the incremental technique was proposed to guarantee the necessary light penetration and to reduce the polymerization shrinkage stress. This technique has been used until today, but it is a delicate procedure that demands a long working time. Thus, alternative formulations were launched on the market [4], but the theoretical advantages did not reflect in clinical benefits. More recently, bulk-fill composites were developed and seem to be occupying good portion of daily clinical use in restorative dentistry [5].
Bulk-fill resin-based composites were developed to allow the operator to sculpt with greater ease and shorter working time due to the insertion of larger increments in the cavity [5,6]. However, despite the several advantages related to such composites, it is necessary to understand how they behave when used in clinical scenarios of extremely deep cavities. Several studies have already been carried out with bulk-fill composites, but usually considering increments of a maximum of 4 or 5mm in depth, which differs from several clinical situations that dental surgeon faces in clinical routine as for example in cases of restorations involving proximal posterior teeth walls or even when it is necessary to make a filling core in endodontically treated teeth [5,7,8].
In general, bulk-fill composites were initially presented in formulations with a high translucency degree so that there would be an adequate passage of light emitted by the light source and consequently a satisfactory activation of the increment [9]. However, the high aesthetic demand has made manufacturers to develop materials with a greater opacity degree [10,11].
As consequence, questions arise about the possibility of adequate activation of these increments, as the more opaque materials tend to block the passage of light. Furthermore, the studies with bulk-fill resins usually consider the use of light sources of high irradiance, many of them of high cost, which does not reflect the working condition of every clinician [12].
In view of the existing doubts to date, the present study aimed to determine the influence of the operative technique (conventional or bulk-fill), material’s opacity and the light sources on the curing potential (CP) in deep cavities. The research hypothesis was that bulk-fill composites have a superior polymerization capacity to the incremental technique regardless of the materials’ opacity and the light curing unit.
MATERIALS AND METHODS
Materials used
The current investigation used two bulk-fill composites of regular viscosity (Opus - FGM and Filtek One - 3M) and a conventional one for the incremental technique (Sirius Z - DFL). Table I shows the materials’ formulations described by the manufacturers.
Table I - Resin composites used in the current study
Characterization of materials’ opacity
To quantitatively determine the opacity level of each material, disk-shaped samples (n = 3) were made in a Teflon-based mold (10mm in diameter and 1mm in thickness). After 24 hours of dry and dark storage, the samples were placed on white (L* = 93.7, a* = 1.2, b* = 0.8) and black (L* = 8.6; a* = - 0.7; b* = - 1.5) standardized backgrounds for readings with spectrophotometer (SP60 X-Rite, Grand Rapids, Michigan, EUA) in reflection mode to determine L*, a*, b* parameters, where L* represents the luminosity (100 is white and 0 is black), a* and b* represent the red-green and yellow-blue chromaticity coordinates, respectively. Thus, opacity was determined by the translucency parameter (TP), which was calculated using the formula described below:
where “w” refers to those values measured on the white background and “b” on the black. The equipment was previously calibrated in its calibration standard before each sample reading.
Light sources
Considering the purpose of the study, two light sources based on LEDs were considered: Radii-Cal (SDI, Bayswater, Victoria, Australia) and Bluephase G2 (Ivoclar Vivadent, Liechtenstein). The radiant exitance of each source was determined by dividing the power - determined in mW by a power meter (Ophir Optronics Solutions, Jerusalem, Israel) - by the emission area of the light source (in cm2). Readings were performed with the LCU tip directly positioned on the power meter’s sensor and also with a 8 mm-thickness spacer.
Degree of conversion
The degree of conversion was determined by Fourier-transformed infrared spectroscopy with an attenuation reflectance accessory (ATR-FTIR, Alpha, Bruker Optics, Ettlingen, Germany). Readings were taken at every 4 mm-thickness increment when bulk-fill composites were used and every 2mm-thickness increment when the conventional composite was considered. For spectral analysis, the vibrating modes of the carbon chains in the peaks of ~1638 cm-1 (aliphatic bonds) and ~1608 cm-1 (aromatic bonds) were considered before and after the photoactivation process, considering the manufacturers’ recommended light exposure times. Thus, the degree of conversion (DC, in %) was determined by the formula:
Statistical analysis
One-way analysis of variance (ANOVA) and Tukey’s tests (95% significance) were applied for those results of opacity. The results of the degree of conversion obtained from the FTIR analyses were submitted to two-way ANOVA (light curing unit and depth) and the Tukey’s test (95% significance). Independent analyzes were performed for each material tested.
Pearson’s correlation tests (95%) were used to verify the potential relationship between material’s opacity and the loss of conversion as a function of depth. Independent analyzes were used for each light source tested.
RESULTS
Table II illustrates the values found for the opacity degree of each material and it is possible to observe that Sirius-Z was the most translucent material and Filtek One the most opaque. Opus BF presented intermediate values.
Table III illustrates the radiant exitance values obtained by the light curing units. According to data taken from the LCU tips close to the power meter sensor, there was no great difference between the results obtained, with Bluephase G2 higher than Radii-Cal. However, when considering the outcomes observed with light sources with 8mm spacing it is possible to see a huge discrepancy, with the Bluephase G2 showing a higher result than Radii-Cal (≅55% higher).
Figure 1 details the degree of conversion as a function of depth and LCU for Sirius-Z resin composite, where there were no significant differences as a function of depth and LCU. The degree of conversion at the bottom of the cavity as a function of light exposure by the incremental technique is shown in Figure 2. Although the Radii-Cal source promotes lower initial values, the degree of conversion is increased as new light exposures are performed with subsequent increments.
Figure 3 presents the degree of conversion as a function of depth and LCU for Opus BF resin composite, where in the top region had no difference in the degree of conversion depending on the light source used, while in the base region the light source Radii-Cal produced lower conversion values. It was observed that, in both light sources, the degree of conversion of the base was lower than the one produced at the top. The average conversion values of the Opus BF resin at the bottom of the cavity as a function of light exposure are shown in Figure 4. As can be seen, additional light exposure resulted in the largest discrepancy in values produced by the tested light sources.
In Figure 5, the values of the Filtek One resin were presented, where the top results had little difference, while the base values were higher in the Bluephase. The degree of conversion of the base was slightly higher in the Bluephase light source and in the Radii-Cal light source the degree of conversion of the base was lower when compared to the top. Figure 6 shows the degree of conversion of Filtek One resin to the bottom of the cavity as a function of exposure to light. Despite the degree of conversion being higher with the exposure to the second increment for both light sources, the superiority of the Bluephase G2 source was maintained.
The loss of conversion percentage due to depth and the source of light employed is shown in Figure 7. It can be seen that for the incremental technique with Sirius-Z resin there was no loss of conversion, regardless of the light source employed values were higher than those verified for the most superficial region. Regarding bulk-fill resins, it can be seen that the loss of conversion as a function of depth was dependent on the light source used. Figures 8 and 9 show the relationship between opacity and loss of conversion due to depth when using the Radii-Cal and Bluephase G2. When considering the correlation coefficients determined by the Pearson test, it appears that the correlation is significant when using the Radii-Cal source, but the same was not evidenced when using Bluephase G2. There are additional details about direct restoration techniques that you can obtain on our course "International schools working with composite restorations".
Table II - Opacity values for each material tested
Table III - Radiant exitance values emitted by light sources
Figure 1 - Degree of conversion as a function of depth and LCU for Sirius-Z resin composite.
Figure 2 - Degree of conversion at the bottom of the cavity as a function of light exposure when using the incremental technique (Sirius Z).
Figure 3 - Degree of conversion as a function of depth and LCU for Opus BF resin composite.
Figure 4 - Degree of conversion at the bottom of the cavity as a function of light exposure when using the bulk fill technique with Opus BF.
Figure 5 - Degree of conversion as a function of depth and LCU for Filtek One resin composite.
Figure 6 - Degree of conversion at the bottom of the cavity as a function of light exposure when using the bulk fill technique with Filtek One.
Figure 7 - Degree of conversion loss ratio according the materials’ and LCUs tested.
Figure 8 - Relationship between materials’ opacity and degree of conversion loss ratio when using Radii-Cal LCU.
Figure 9 - Relationship between materials’ opacity and degree of conversion loss ratio when using Bluephase G2 LCU.
DISCUSSION
The simplicity of bulk-fill technique arouses great interest from a clinical perspective due to the potential working time reduction [13,14]. However, it is foreseeable that questions arise regarding the effectiveness of the polymerization and whether it is associated with the translucency degree of the material used in deep cavities. Thus, the current research determined the influence of the operative technique and the light curing unit on the degree of conversion due to the depth of bulk-fill composites with different translucency degrees. The research hypothesis - that bulk-fill composites have a superior polymerization capacity to the incremental technique regardless of the commercial brand and light source employed – was rejected.
In order to increase the depth of polymerization, approaches in the literature report that in order to obtain greater translucency and guarantee the adequate passage of light throughout the increment, the amount of charge particles is reduced and the size of these particles increased [15]. In addition, the relationship between light filling and dispersion matrix is reduced so that light can penetrate deeper areas [16]. Some manufacturers claim that to the monomer to be fully converted to polymer, even if inserted in 4mm increments and with an acceptable opacity value, alternative photoinitiator systems can be added [6], as the APS system employed in Opus bulk-fill APS resin. However, this same material has greater translucency and it cannot be said that the effectiveness of polymerization is associated with the initiation system. In the opposite Filtek One becomes more opaque during photoactivation, although they are highly translucent at the beginning of activation. As the quality of activation in deeper layers is directly influenced by the number of photons that reach these areas, an improved opacity during polymerization can negatively affect the kinetics of photoactivation and the degree of conversion in deeper layers [17]. Indeed, the current outcomes confirm that this material presented the highest opacity within the tested composites.
Two light sources were chosen as representatives of possible scenarios to be observed in clinical practice. One of them is quite popular within clinicians (Radii-Cal) and the other one (Bluephase G2) is known to be a powerful light source with a great capacity for activation, as demonstrated by previous publications [18-20]. In the present study, Radii-Cal emitted and Bluephase G2 presented similar when considered those readings taken close to the power meter sensor, but high discrepancy as the light source was moved 8mm away. The difference can be caused by factors such as varied beam profiles or active tip diameter. It should be considered that the Radii-Cal device has a LED protective lens, an acrylic part adapted to the device’s tip and which causes an increase in its diameter, a factor that can significantly influence the amount of light dissipated and capable of reach the bottom of thick restorations [20].
The degree of conversion considers the amount of monomers converted into polymers and therefore is associated with the resultant physical-mechanical properties, such as color stability, surface hardness, fracture resistance and others. Thus, a low conversion can be resultant from free monomers, which may be leached in the presence of oral cavity moisture and accelerate the degradation process of resin-based composite and compromise its durability [21,22]. Therefore, it is essential that the activation process occurs efficiently.
Regarding the incremental technique, the degree of conversion as a function to depth (Figure 1) was not affected by the light curing unit. Although Radii-Cal presents lower radiant exitance than Bluephase G2, the overexposure of light in multiple increments appears to attenuate the differences arising from the light curing devices. Furthermore, most increments of conventional resins become progressively translucent during polymerization, which allows an improved light transmission in depth during the activation of the increment [15,17,20,23].
The degree of conversion as a function of depth followed a similar trend for Opus BF (Figure 3) and Filtek One (Figure 5), showing stability for those values obtained by Bluephase G2 and significant differences with Radii-Cal – where those final values found at the bottom were considerably lower than that from the top. These results are explained by its radiant exitance emitted when at a distance of 4mm from the increment. The Bluephase G2 showed a great increase in the degree of conversion due to light exposures (Figures 4 and 6), which can be explained by its high radiant exitance and low light dispersion when at a distance of 4mm from the increment.
Considering the overall study, the incremental technique did not result in loss of conversion due to depth or light source (Figure 7). Indeed, the final conversion at 8mm depth showed was even higher (numerically) as that of the top as it receives a greater number of activation exposures, regardless of the photoactivating unit used. In contrast, Opus BF resin had loss of conversion in both light source units, being the loss with Radii-Cal considerably greater than that produced by Bluephase G2. Bluephase G2 showed an insignificant loss of conversion when used to activate the Filtek One resin, while the Radii-Cal apparatus showed a significant loss of conversion. Therefore, it is possible to identify an association between the values obtained when related to opacity with loss of conversion due to depth (Figure 8) using the Radii-Cal, showing a correlation coefficient r = 0.891707246. On the opposite, this association was not seen when using the Bluephase G2 curing device (Figure 9), indicating a correlation coefficient r = 0.515703768.
CONCLUSIONS
The research hypothesis - that bulk-fill composites have a superior polymerization capacity to the incremental technique regardless of the materials’ opacity and the light curing unit – was rejected.
Depending on the light curing, the bulk-fill technique can compromise the curing potential in deep cavities.
The effect of materials’ opacity was dependent on the light curing unit used.
If you enjoyed reading this article and would like to explore the composite restoration treatment protocols fu, we encourage you to enroll our course "Brazilian School of Aesthetic Restorations".
List of authors:
Lorena Aparecida Nery Araujo, Larissa Maria Cavalcante, Luis Felipe Schneider
References
Ferracane JL. Resin composite: state of the art. Dent Mater. 2011;27(1):29-38.
Villarroel M, Fahl N, De Sousa AM, De Oliveira OB Jr. Direct esthetic restorations based on translucency and opacity of composite resins. J Esthet Restor Dent. 2011;23(2):73-87.
Hamlin NJ, Bailey C, Motyka NC, Vandewalle KS. Effect of tooth-structure thickness on light attenuation and depth of cure. Oper Dent. 2016;41(2):200-7.
Bacchi A, Feitosa VP, da Silva Fonseca AS, Cavalcante LM, Silikas N, Schneider LF. Shrinkage, stress, and modulus of dimethacrylate, ormocer, and silorane composites. J Conserv Dent. 2015;18(5):384-8.
Chesterman J, Jowett A, Gallacher A, Nixon P. Bulk-fill resin-based composite restorative materials: a review. Br Dent J. 2017;222(5):337-44.
Shimokawa CAK, Turbino ML, Giannini M, Braga RR, Price RB. Effect of light curing units on the polymerization of bulk fill resin-based composites. Dent Mater. 2018;34(8):1211-21.
Van Ende A, De Munck J, Lise DP, Van Meerbeek B. Bulk-fill composites: a review of the current literature. J Adhes Dent. 2017;19(2):95-109.
Reis AF, Vestphal M, Amaral RCD, Rodrigues JA, Roulet JF, Roscoe MG. Efficiency of polymerization of bulk-fill composite resins: a systematic review. Braz Oral Res. 2017;31(Suppl. 1):e59.
Pereira LDE, Couto MP No, Pereira RG, Schneider LFJ. Influence of resin matrix on the rheology, translucency, and curing potential of experimental flowable composites for bulk-fill applications. Dent Mater. 2021;37(6):1046-53.
Ilie N. Sufficiency of curing in high-viscosity bulk-fill resin composites with enhanced opacity. Clin Oral Investig. 2019;23(2):747-55.
Farahat F, Davari A, Fadakarfard M. Effect of storage time and composite thickness on degree of conversion of bulk-fill and universal composites using FTIR method. Braz Dent Sci. 2020;23(2):1-6.
Omidi BR, Gosili A, Jaber-Ansari M, Mahdkhah A. Intensity output and effectiveness of light curing units in dental offices. J Clin Exp Dent. 2018;10(6):e555-60.
Vianna-de-Pinho MG, Rego GF, Vidal ML, Alonso RCB, Schneider LFJ, Cavalcante LM. Clinical time required and internal adaptation in cavities restored with bulk-fill composites. J Contemp Dent Pract. 2017;18(12):1107-11.
Tardem C, Albuquerque EG, Lopes LS, Marins SS, Calazans FS, Poubel LA, et al. Clinical time and postoperative sensitivity after use of bulk-fill (syringe and capsule) vs. incremental filling composites: a randomized clinical trial. Braz Oral Res. 2019;33:e089.
Bucuta S, Ilie N. Light transmittance and micro-mechanical properties of bulk fill vs. conventional resin based composites. Clin Oral Investig. 2014;18(8):1991-2000.
Ilie N, Bucuta S, Draenert M. Bulk-fill resin-based composites: an in vitro assessment of their mechanical performance. Oper Dent. 2013;38(6):618-25.
Ilie N. Impact of light transmittance mode on polymerization kinetics in bulk-fill resin-based composites. J Dent. 2017;63:51-9.
SoaresCJ, Rodrigues MP, Oliveira LRS, Braga SSL, Barcelos LM, Silva GRD, et al. An evaluation of the light output from 22 contemporary light curing units. Braz Dent J. 2017;28(3):362-71.
Shimokawa CA, Turbino ML, Harlow JE, Price HL, Price RB. Light output from six battery operated dental curing lights. Mater Sci Eng C Mater Biol Appl. 2016;69:1036-42.
Schneider LFJ, Carmello AC, Pereira LE, Couto MP No, Modena RA, Cavalcante LM. Influence of light-curing units on the activation potential of bulk-fill composites. J Clin Dent Res. 2019;16:128-41.
Ferracane JL, Berge HX, Condon JR. In vitro aging of dental composites in water--effect of degree of conversion, filler volume, and filler/matrix coupling. J Biomed Mater Res. 1998;42(3):465-72
Arruda BM, Bassi JC, Vitti RP, Scatolin RS. Color stability of bulk fill composite resins submitted to coffee staining. Braz Dent Sci. 2021;24:1-7.
Harrington E, Wilson HJ, Shortall AC. Light-activated restorative materials: a method of determining effective radiation times. J Oral Rehabil. 1996;23(3):210-8.
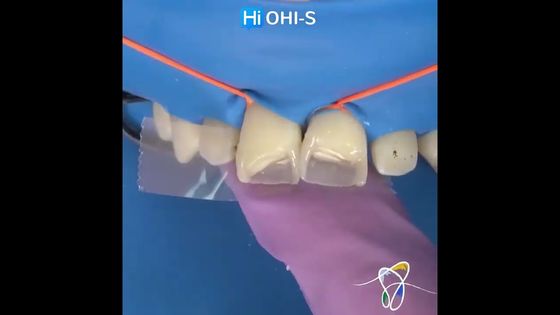
Video
OHI-S
02 April 2024
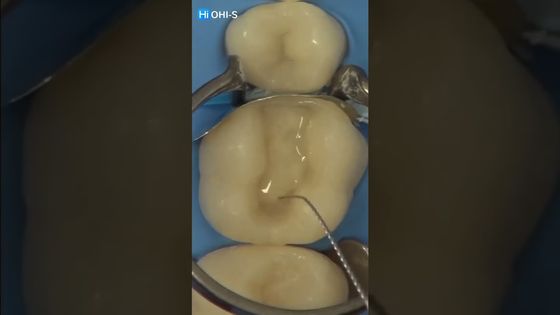
Video
OHI-S
16 January 2024
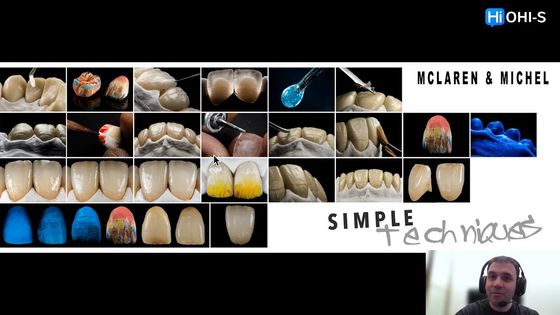
Video
OHI-S
21 November 2023