Resin Infiltration of Non-Cavitated Enamel Lesions in Paediatric Dentistry: A Narrative Review
Abstract: The resin infiltration (RI) technique was introduced as one of the minimal intervention dentistry strategies in addressing dental caries among the paediatric population. This technique used the low-viscosity resin monomer to infiltrate the non-cavitated carious lesion and other developmental enamel porosities, thus allowing the conservation of the tooth structure. This narrative review aims to explore the value of RI in Paediatric Dentistry. Through our search of the literature, the development of the material, their clinical applications and shortcomings, as well as the innovation that has been carried out to improve the current RI, were discussed. There are number of high-level evidence supporting the use of RI in arresting non-cavitated proximal caries lesions in primary and permanent teeth, but its efficacy in managing anterior white spot lesions is still unclear. Limited penetration depth, not radiopaque and questionable long-term colour and material stability were among the limitation of the material. Various laboratory-based studies have been conducted to improve the current properties of RI. Nevertheless, RI has emerged as one of the important micro-invasive techniques in addressing non-cavitated and anterior white-spot enamel lesions in children and adolescents with great success.
1. Introduction
Managing dental caries among the paediatric population is a never-ending story. Although dental caries is preventable, it has affected more than 600 million children worldwide [1], and without prompt intervention, the disease can progress and increase the morbidity of the child [2]. With a better understanding of the etiological factors and behaviour of dental caries, the concept of caries management in the modern era has now changed [3]. The minimal intervention dentistry (MID) concept has been introduced, emphasising early detection of dental caries and the promotion of preventive therapy. Advancement of the lesion should be managed by adopting the minimally invasive operative approach [4]. Thanking Buonocore [5] and Bowen [6] for their discovery of the acid etching technique and the bisphenol A-glycidyl methacrylate (Bis-GMA) resin monomer, respectively, various resin-based dental materials that support the MID concept have been developed.
Resin infiltrant (RI), marketed as Icon® (DMG, Hamburg, Germany), is among the products that benefited from Buonocore’s and Bowen’s breakthrough discovery. The RI technique has emerged as one of the important tools that support the MID concept in managing non-cavitated carious lesions, using the tagline ‘Drilling? No thanks!’. This novel material uses the concept of infiltrating the caries lesion using a low-viscosity resin in an attempt to arrest the progression of the non-cavitated caries lesion without the need of removing the tooth structure. This concept is highly desirable and well appreciated, especially in the field of Paediatric Dentistry, since the drilling procedure may not be well-tolerated by young and highly anxious children [7].
This narrative review article aims at exploring the usage of the RI technique in Paediatric Dentistry. We divided the paper into three sections: the past, where we revisited the early development of the RI; the present, where we discussed the current utilisation of the techniques in paediatric patients and their shortcomings and the future, where the innovation and improvisation that has been made to the current RI formulation were explored.
2. The Past: Development of RI
2.1. The Resin Monomer
Surprisingly, the idea of infiltrating early caries lesions has existed for more than five decades. Researchers since the 1970s have conducted many in vitro and in vivo studies to find the right material that is able to infiltrate the early enamel lesion effectively [8–13].
Going back to 1975, Davila and colleagues [8] investigated the potential ability of adhesives to seal early enamel lesions. They proposed an idea that the demineralised surface of the lesion would act as a suitable locus for the adhesives to infiltrate, sealing the lesion entrance and spaces, thus arresting the ongoing demineralisation and preventing cavity formation. They managed to infiltrate the artificial caries lesion with adhesives in vitro with deeper penetration depth seen in the lesions pre-treated with acid conditioning [8].
A year later, Robinson et al. [9] used an experimental resorcinol-formaldehyde resin to occlude the pores of early enamel lesions. The resin occluded up to 90% of the pores after the second and third time of applications and simultaneously reduced the progression of the demineralisation. However, due to the potential cytotoxic hazard of the resin, mainly towards the vital dentine and pulp tissue, the use of the resin was discontinued [9,10]. The authors later suggested that the infiltrating resin should meet a certain standard: low viscosity, hydrophilic, anti-bacterial, non-toxic, aesthetically acceptable and able to polymerise into a solid state. The latter recommendation may give extra strength to the weakened enamel structure following demineralisation [10].
These two early experiments have opened the ‘floodgates’ to various in vitro and in vivo studies focusing on developing resins that can infiltrate and halt the progression of early enamel lesions. Earlier studies have used unfilled resin in the form of dental adhesives and sealants as the infiltrating agent. In vitro studies on an artificial caries model shows some potential, where all of the studies reported a reduction in caries progression as compared to the control [11–13]. In one study by Robinson et al. [11], they observed that 60% of the pores were occluded following the infiltration process.
Clinical evaluations on the success of sealing the enamel lesion using sealants and adhesives among children were carried out by Gomez et al. [14] and later Martignon et al. [15]. While the latter reported a significantly reduced but still relatively high lesion progression (43.5%) over 18 months of observation, the former found no significant difference between the infiltrated and control lesion in their 24-month clinical study. It was suggested that the findings might be due to the disintegration of the material over time or inadequate sealing of the lesion [14]. This reflects the inability of the sealants and adhesives to penetrate deep into the lesion and, therefore, only showed superficial penetration into natural enamel lesions [16].
This led to a series of in vitro and in vivo studies led by Meyer-Lueckel and Paris [17,18] to develop a new material that does not disintegrate easily, has a good sealing ability and is able to penetrate deep into the base of the porous enamel lesion. The penetration ability of the material into a porous structure is best explained by theWashburn equation [19]. Based on the equation, the porous solid (in this context, the enamel structure) was regarded as a structure consisting of a collection of open capillaries, which can be penetrated by liquids driven by the capillary forces. Further, a material with high penetration coefficient (PC) can penetrate deeper and faster into the porous structure [20] and, upon hardening, can directly occlude and seals the porosity [17].
An earlier experiment conducted by Meyer-Lueckel and Paris [17] showed that materials with PC of more than 100 cm/s might be more suitable for application as ‘infiltrants’ for the treatment of early enamel lesions as compared to fissure sealants and adhesives. Furthermore, the same authors also found that resin materials with high triethylene glycol dimethacrylate (TEGDMA) concentrations tended to show better inhibition of lesion progression. This effect can probably be attributed to the better penetration capabilities of TEGDMA-based resins [17].
Their experimental resins using a mixture of TEDGMA, 2-hydroxyethyl methacrylate (HEMA), and ethanol showed the highest PC value, attributed to their low contact angle and low viscosity of these components. However, the mixture consisting of HEMA and ethanol showed inadequate hardening and was thus deemed unsuitable for infiltration of porous enamel. The mixture of resin monomer TEDGMA and ethanol, on the other hand, showed high PC value and sufficient hardening [18], suggesting that these two components would be the benchmark for the future development of RI.
Further studies on the development of RI were based on the different formulations of TEDGMA and ethanol. Paris et al. [18] found that 99% of TEDGMA-containing resin gave a PC value of 204 cm/s, while the addition of TEDGMA and ethanol as solvents gave a PC value of 391 cm/s. The addition of solvent has been shown to increase the penetration coefficient and, consequently, the penetration depths, but inhomogeneities and uncured areas within the resin layer may occur, which can lead to incomplete polymerisation. Therefore, the authors suggest that the addition of ethanol to increase penetration abilities should be carefully balanced with the potentially impaired properties of the cured material.
They later concluded that low-viscosity RIs, based mainly on TEGDMA, had relatively high PC and were capable of inhibiting the progression of both artificial [21] and natural enamel caries lesions [22]. The high PC values also allow the material penetrates deep into the lesion body in a low-demineralising environment [23,24], and a 3 min application is sufficient to completely occlude the pores of the early enamel lesion [25].
2.2. Etching
As described by theWashburn equation, the infiltration of resin into the porous enamel lesion is driven by capillary forces. The infiltration of the resin is strongly influenced by both the pore volume and the capillary radius of the solid to be penetrated. In the case of infiltrating early caries lesions, the presence of the highly mineralised surface layer with low pore volume might prevent the penetration of the infiltrating resin into the subsurface porosities underneath it [26]. Elimination of the surface layer to expose the porous subsurface lesion is desirable to aid the penetration of the resin. To achieve this, pretreatment conditioning of the surface layer is needed prior to the infiltration process [16].
In restorative dentistry, acid etching has been identified as the core step in pretreatment conditioning of the enamel surface prior to the placement of resin composite. Etching the enamel with 30–40% phosphoric acid resulted in selective demineralisation of the enamel prisms of varying irregularities and microporosities [27]. This allows the lowviscosity resins to ‘penetrate’ the microporosities, forming resin tags and micromechanical interlocking between the resins and the enamel [28]. Inspired by the success of the acid etching technique, a similar application might work for RI.
Grey and Shellis [29] successfully etched the artificial enamel caries with 36% phosphoric acid, which improved the penetration of the resin into the subsurface enamel lesion. Their in vitro results, however, cannot be replicated in the actual clinical scenario. Meyer-Lueckel et al. [30] found out that the penetration depth of the resin into the enamel porosities between the artificial and natural caries lesion was different. They postulated that the mineral content of the surface layer in natural caries lesions might be higher and more inhomogeneous due to the continuous cycles of de- and remineralisation in the oral cavity. They later concluded that complete erosion of the surface layer is necessary to expose the subsurface lesion prior to infiltration with low-viscosity resins.
Two in vitro studies carried out by Meyer-Lueckel, Paris and their team [30,31] have demonstrated that in comparison to 37% phosphoric acid and 5% hydrochloric acid, the use of 15% hydrochloric acid gel for 90–120 s was effective in not only eroding the surface layer but led to nearly complete removal of the structure. This leads to exposure of the subsurface enamel lesion, which is ready to be infiltrated by the resin. However, without pre-treatment of the surface layer, penetration of resin was limited [29] and almost impossible for it to reach the subsurface enamel lesion.
Elimination of the surface layer also can be performed mechanically, for instance, by using a diamond bur or polishing strip [32]. However, this procedure would cause occlusion of the lesion pores by smear layer, which later will affect the infiltration process of the resins. Furthermore, the reduction of the surface layer mechanically is difficult to control [30]. Thus, optimum surface erosion seems the best option in allowing the penetration of the low-viscosity resin into the subsurface enamel lesion effectively.
2.3. Ethanol
The use of ethanol as a solvent is believed to reduce the viscosity of the penetrating resin, as well as remove the remaining residual water that might be present at the bottom of the body lesion. Meyer-Lueckel et al. [16] found that the addition of ethanol enhanced the penetration ability of dental adhesives. Similar results were found with the experimental resin that they developed. The addition of 10% and 20% of ethanol had shown to increase the PC as well as the penetration depth [17,18].
Therefore, it can be assumed that a solvent like ethanol might affect the penetration behaviour positively. Alas, there is concern regarding the addition of the evaporating solvent that might possibly cause inhomogeneities and incomplete polymerised areas within the resin itself [18]. Paris et al. [33] conducted an in vitro study on extracted primary molars using experimental resins with and without the addition of ethanol. Although the PC value of ethanol-containing resin is higher, both the solvent-free and ethanolcontaining resins managed to infiltrate the lesion completely. Hence, the authors suggest that concerning the potential effect of ethanol on the polymerisation of the RI, a solvent-free infiltrant is preferable [33]. In order to facilitate the removal of residual water and protein within the body lesion, the commercially available RI add-ons another step: pre-treatment of the enamel surface with ethanol following the acid etching procedure.
A summary of the main studies related to the development of the current RI is shown in Table 1.
Table 1. Summary of main studies related to the development of RI.
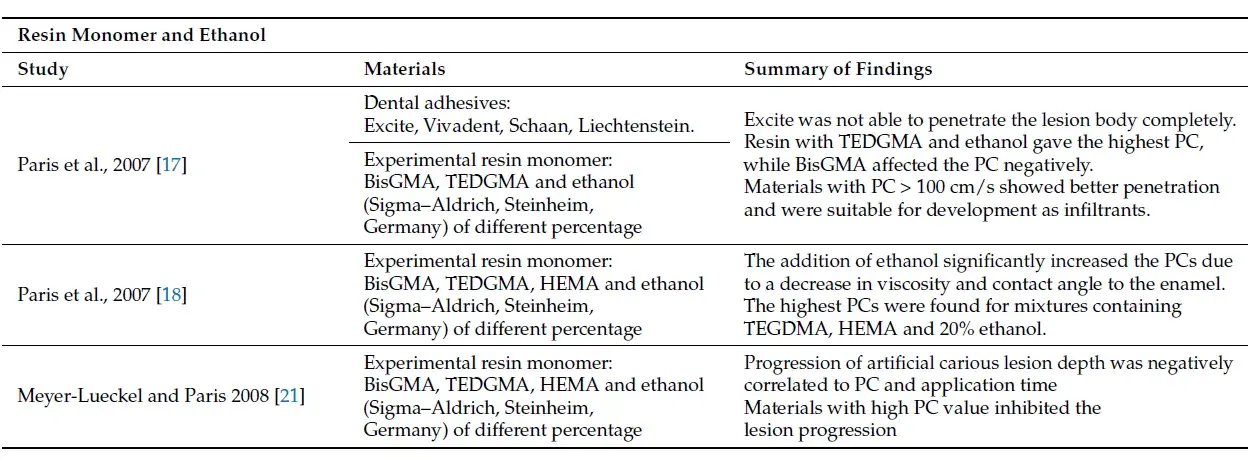
3. Present: Clinical Usage and Limitations of the Current RI
3.1. Arresting Non-Cavitated Early Enamel Lesion
The ultimate aim of developing RI is to delay the restorative procedure on a noncavitated early enamel lesion. Placement of restoration will irreversibly remove the sound tooth structure surrounding the lesion and initiates the so-called ‘restorative cycles’ [34]. The use of fluoride varnish and casein phosphopeptide-amorphous calcium phosphate (CPP-ACP), coupled with good oral hygiene practise has been the mainstream in managing the non-cavitated early enamel lesion previously [35,36]. However, the remineralisation process requires time to be accomplished and is not immediate, highly dependent on the patient’s compliance and good oral hygiene measures [37] and unable to completely mask the white spot lesion [38,39].
RI, on the other hand, infiltrates the early enamel lesion using the low-viscosity resin monomer. The concept of this technique is based on the understanding of the structure and demineralisation process of the early enamel lesion itself. Following the demineralisation of enamel under an acidic challenge, minerals are dissolved out of the enamel, creating porosities within the enamel structure. These porosities act as a diffusion pathway for the dissolved minerals and acids. Infiltrating the early enamel lesion will occlude the pores and seals the lesion inside instead of at the surface. This will block the diffusion pathway of the organic acids, thus arresting the caries progression and inhibiting future demineralisation [17]. Further, infiltration also improves the mechanical strength of the porous enamel [24], resisting future demineralisation.
The clinical success of infiltrating early enamel lesions is evident in the literature, both for primary and permanent teeth. Infiltrating the non-cavitated early enamel lesion on the proximal surface has been the subject of many clinical trials. Ekstrand et al. [40], Page et al. [41] and Ammari et al. [42] reported a significant reduction in caries progression of the lesions on primary molars after infiltration with resin as compared to fluoride therapy. The therapeutic effects of RI over the fluoride varnish application were 21% higher over a two-year study period [41]. In addition, Urquhart et al. [43] reported that a combination of both RI and the application of 5% fluoride varnish on proximal early enamel lesions were five times more likely to cause caries arrest as compared to no treatment. Similar success was also reported in clinical trials on proximal lesions of permanent teeth, favouring the use of RI over fluoride therapy in managing non-cavitated proximal enamel lesions [44–46].
The performance of RI on the occlusal surface of teeth also has been investigated. Bakshandeh and Ekstrand [47] conducted a split-mouth study on the efficacy of RI in sealing the occlusal early enamel lesion of the primary molar tooth. Following an observational period of 22 months, the application of RI in combination with fluoride varnish significantly reduced the lesion progression as compared to the application of fluoride varnish alone. Later, Barakat and El-Soud [48] conducted another split-mouth study on children comparing the effectiveness of RI and compomer as a pit and fissure sealant material. They concluded that infiltration of the occlusal surface with resin provides a better retention rate as compared to compomer but harbours more bacteria due to the fluoride-releasing ability of the latter material.
The usage of RI in non-cavitated occlusal and proximal early enamel lesions is endorsed by the American Dental Association [49] in their evidence-based clinical practice guideline published in 2018. The guideline recommends the use of RI alone or in a combination of 5% fluoride varnish in arresting and reversing the non-cavitated lesion on the proximal surface, whereas for the lesion on the occlusal surface, a combination of RI and 5% fluoride varnish can be considered as an alternative to the conventional treatment of using fluoride varnish or fissure sealant. They believed that the relatively high cost of RI, as compared to sealants, might be the deterrent factor for its widespread use in community-based dentistry.
Further, two recently published systematic [50] and umbrella [51] reviews on the caries inhibition ability of RI on early enamel lesions showed that the relative risk for caries progression for the caries-infiltrated lesion is significantly reduced. Infiltrating the caries lesion is more effective for both primary and permanent teeth as compared to no treatment or other non-invasive preventive measures such as fluoride varnish, fissure sealant and oral hygiene instruction [50,51]. The authors also pointed out that the comparison of RI efficacy and caries lesion progression on primary and permanent teeth favours the successors, mainly attributed to the structural difference of enamel between the primary and permanent teeth. Lesion progression is quicker in primary teeth due to the fact that the enamel structure is less mineralised, more porous and thinner [50] as compared to permanent teeth.
Table 2 summerises the main studies on the ability of RI to arrest non-cavitated early enamel lesions.
Table 2. Summary of main studies on the ability of RI to arrest non-cavitated early enamel lesions.
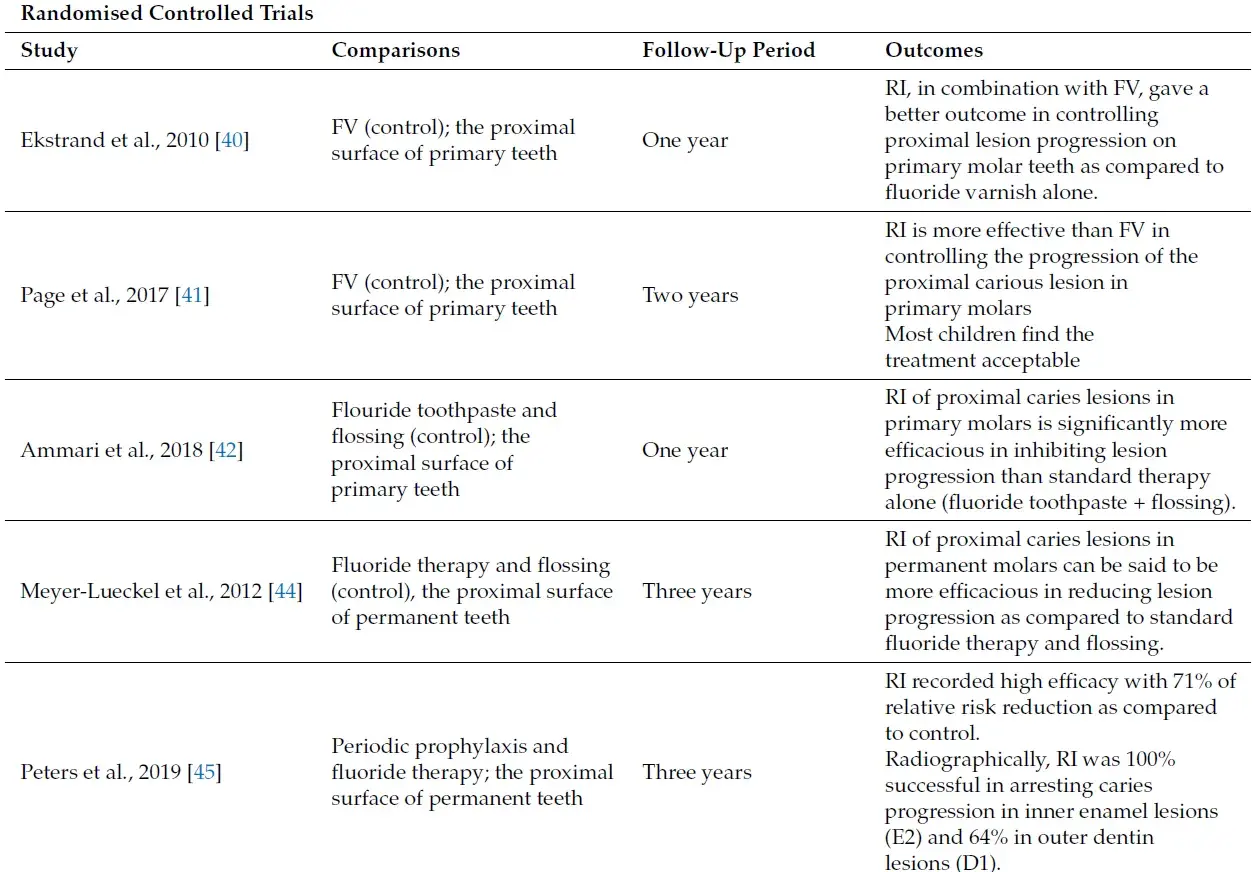
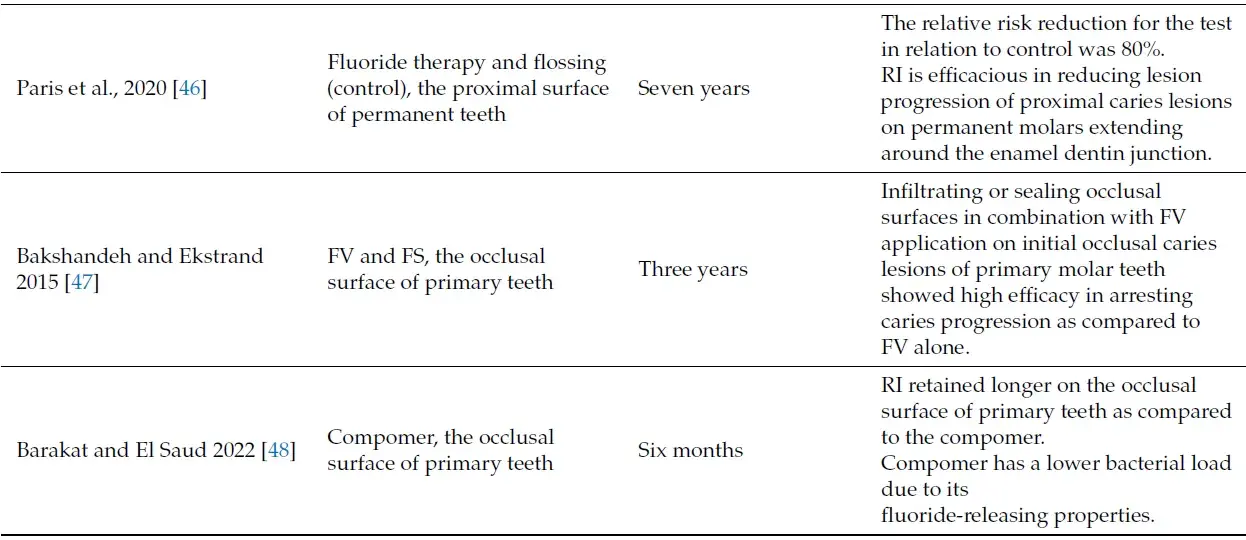
3.2. Aesthetic Improvement of the Anterior White-Spot Lesion (WSL)
The ability of RI to infiltrate the non-cavitated early enamel lesion offers a new pathway and treatment modality in managing enamel WSL on the anterior teeth. The presence of the WSL is a result of enamel structure porosities, either due to enamel decalcification following the caries process or developmental disturbance during enamel formation. Clinically, the WSL appears as a chalky, whitish opacities surrounded by a normal translucent enamel due to the difference in optical density, measured as a refractive index between the normal and the porous enamel [52]. The subsurface demineralisation of the porous enamel retains more air (refractive index = 1.0) and water (refractive index = 1.33), causing higher light scattering as compared to the solid enamel structure (refractive index = 1.62). This led to a lower refractive index value that contributed to the abovementioned appearance. Poor aesthetic appearance [53], low self-esteem and negative psychosocial impact [54] have been reported in relation to the presence of WSL on anterior teeth among children.
Post-orthodontic decalcification is commonly associated with the presence of WSL on anterior teeth [55], where it increases the prevalence of WSL by three-fold as compared to those who were not wearing the appliance [56]. The presence of the orthodontic bracket and the archwire might hinder routine oral hygiene care and requires meticulous effort by the patient to maintain the area clean. Retention of dental plaque elevates the cariogenic bacterial count in the saliva, causing depletion in pH value at the dental plaque-enamel interface, thus initiating mineral loss from the enamel structure leading to enamel subsurface porosities [57,58]. The initiation of enamel decalcification can be seen as early as four weeks post orthodontic bracket placement [59].
Fluorosis and molar-incisor hypomineralisation (MIH) are the two most common developmental anomalies associated with WSL formation. Fluorosis occurs as a result of intoxication of the ameloblast cells caused by an excessive level of fluoride during the maturation stage of amelogenesis. In normal circumstances, the ameloblast alternates between smooth and ruffled-ended ameloblast, removing the matrix protein from the extracellular spaces and promoting enamel matrix mineralisation. However, excessive levels of fluoride disrupt the ameloblast cell function, causing amelogenin protein retention and areas of hypomineralisation within the enamel matrix [60,61]. This creates a subsurface enamel hypomineralisation and porosities, seen as WSL intra-orally.
MIH is a developmental defect characterised by hypomineralisation of systemic origin affecting one to all four first permanent molars and simultaneously might affect the permanent incisors as well [62,63]. A systemic and environmental insult towards the ameloblast during the maturation stage of amelogenesis is thought to be the culprit, leading to the formation of a hypomineralised area within the enamel structure. The exact aetiology of MIH is still unclear, but childhood illness, especially fever, asthma and pneumonia, maternal infection during pregnancy, consumption of amoxicillin during the first year of life, low birth weight and chicken pox infection are among the factors that have been proposed [64–68]. Clinically, the appearance of WSL on MIH incisors varies from creamy-white to yellow-brown opacities with well-demarcated borders [68] and may be accompanied by tooth sensitivity.
Reversal of the decalcification process (in the case of post-orthodontic decalcification) and improvement of the aesthetic value have been the aim of managing WSL on anterior teeth. Improvement in the child’s oral health and quality of life has been reported following the treatment of WSL [69]. Similar to the management of non-cavitated early enamel lesions, fluoride-based materials have been used as the first line of treatment in managing such cases [70]. In addition, microabrasion [71,72] and bleaching [73,74] also has been suggested to improve the aesthetic appearance of the WSL.
However, the masking ability and the outcome of these techniques are inconsistent as the aesthetic appearance remains impaired even after treatment [38,75], while the use of bleaching agents in the paediatric population is somewhat controversial, as the side effects of gingival irritation and sensitivity might not be well tolerated in young children. In Europe, the use of bleaching agents in children is restricted to 0.1% hydrogen peroxide [76], which is far from the effective concentration to produce a remarkable aesthetic improvement [77].
Infiltrating the WSL with resin allows the low-viscosity resin to penetrate the intercrystalline spaces of the hypomineralised and porous enamel. This resulted in an improvement in the refractive index value of the porous enamel (1.52), close to the value of normal enamel [78]. This has dramatically and instantly improved the appearance of the anterior teeth with WSL. High patient satisfaction has been reported following the infiltration of WSL [79,80]. Further, infiltration improves the surface microhardness [81] and shear bond strength while reducing the surface roughness of the infiltrated WSL [82].
In the literature, several systematic reviews concluded that there is inconclusive evidence to strongly support the use of RI in managing WSL on anterior teeth. This is, however, merely due to insufficient high-quality clinical trials rather than the clinical inefficiency of the technique itself. Most of the studies available were either laboratorybased, had a high risk of bias or were short-term studies, thus hindering the authors from making a conclusive recommendation [51,83–85]. All of the authors were in agreement regarding the need to conduct more high-quality clinical trials with long-term follow-ups in the future.
Nevertheless, based on the available clinical trials, positive camouflaging effects were reported on the WSL of anterior teeth treated with RI.Wang et al. [86] reported a favourable aesthetic outcome of RI over fluoride varnish among their orthodontic patients. Further, Knosel et al. [87], in their clinical study on post-orthodontic WSL, stated that the lesion showed significant aesthetic improvement and blended well with the normal enamel, even after a 24-months follow-up. Meta-analysis performed by Borouni and colleagues [85] showed a significantly higher optical improvement following infiltration as compared to the regular application of fluoride varnish. The author also stated that the masking effects of fluoride varnish might take up to six months, in contrast to the immediate masking effect of RI. In addition, two studies have been conducted by Gu et al. [71] and later Shan et al. [72] comparing the effects of RI and microabrasion in treating post-orthodontic WSL. They concluded that both techniques improve the aesthetic outcome of the WSL, but better and longer aesthetic outcomes were observed among the lesions treated with RI.
Treatment of WSL secondary to developmental defects using RI has also been the subject of clinical investigation. Clinical trials conducted by Gugnani et al. [74] reported an effective masking ability of RI on teeth with mild to moderate fluorosis, while Schoppmeier et al. [88] concluded that RIwith increased etching and infiltration time gave significantly better aesthetic outcomes in comparison to bleaching. Gencer and Kirzioglu [89], in another study, concluded that treatment with RI on anterior teeth with fluorosis gave the highest colour changes as compared to fluoride varnish, CPP-ACP and microabrasion. This was in agreement with the clinical study conducted by Zotti et al. [79], as they observed the immediate disappearance of the fluorosisWSL following treatment with RI. Significant aesthetic satisfaction was reported by the patients where the changes remained stable during their one-year follow-up.
A recently conducted systematic review also favours RI over microabrasion and bleaching in the management of fluorosis [90]. The author further suggested a combination of in-house bleaching and RI for a better aesthetic outcome. However, Borouni et al. [85] disagreed with the recommendation. Through their meta-analysis, pre-treatment of the fluorosis teeth with bleaching before infiltration did not give any significant masking effects as compared to infiltration alone. Further, the safety usage of bleaching agents among paediatric patients should be evaluated beforehand to avoid unnecessary iatrogenic damage.
RI was also used in the management of anterior teeth with MIH [91,92]. Earlier studies by Kim et al. [93] found that the MIH teeth treated with RI showed significant colour changes after one week of treatment. However, 40% of the infiltrated teeth remained unchanged. They postulated that the failure was associated with the depth of the lesion, which is beyond the penetration ability of the resin, and the highly mineralised surface layer, which had not been eroded long enough to achieve the desired effect. Repeated application of acids to encourage more surface erosion might be able to improve the infiltration efficacy in such lesions. This is further supported by Somani et al. [94]. They stated that variation in the depth of the lesion plays an important role in deciding which treatment protocol should be chosen for a different type of MIH lesion. RI showed some potential and can be effective in reversing the white opacities, provided that the surface layer has been removed sufficiently.
Elbaz and Mahfous [95] and Bhandari et al. [96] both investigated the effect of RI in mild MIH-affected incisors. Both studies reported the efficacy of the RI technique in masking the opacities and improving the aesthetic appearance of the incisors. The former author also observed the superior ability of the RI technique over 5% sodium fluoride is masking the WSL on incisors with mild MIH. In addition, Hasmun et al. [69] used a combination of microabrasion and RI in managing MIH-affected incisors in 23 children. They used digital clinical images as the tools, comparing the pre-and post-treatment effects after six months. The author observed a significant reduction in brightness and surface areas affected by the opacities. They later concluded that the technique is efficient in reducing the incisor opacities related to MIH.
The European Association of Paediatric Dentistry (EAPD) [68], in their recently updated policy document on best clinical practice in managing MIH, suggested that RI can be considered as one of the treatment options in managing MIH-affected incisors, either alone or in combination with other treatments including microabrasion, external bleaching and direct composite restoration, depending on the severity and depth of the lesion.
The main studies on the ability of RI to improve the anterior WSL are shown in Table 3.
Table 3. Summary of main studies on the ability of RI to improve the anterior WSL.
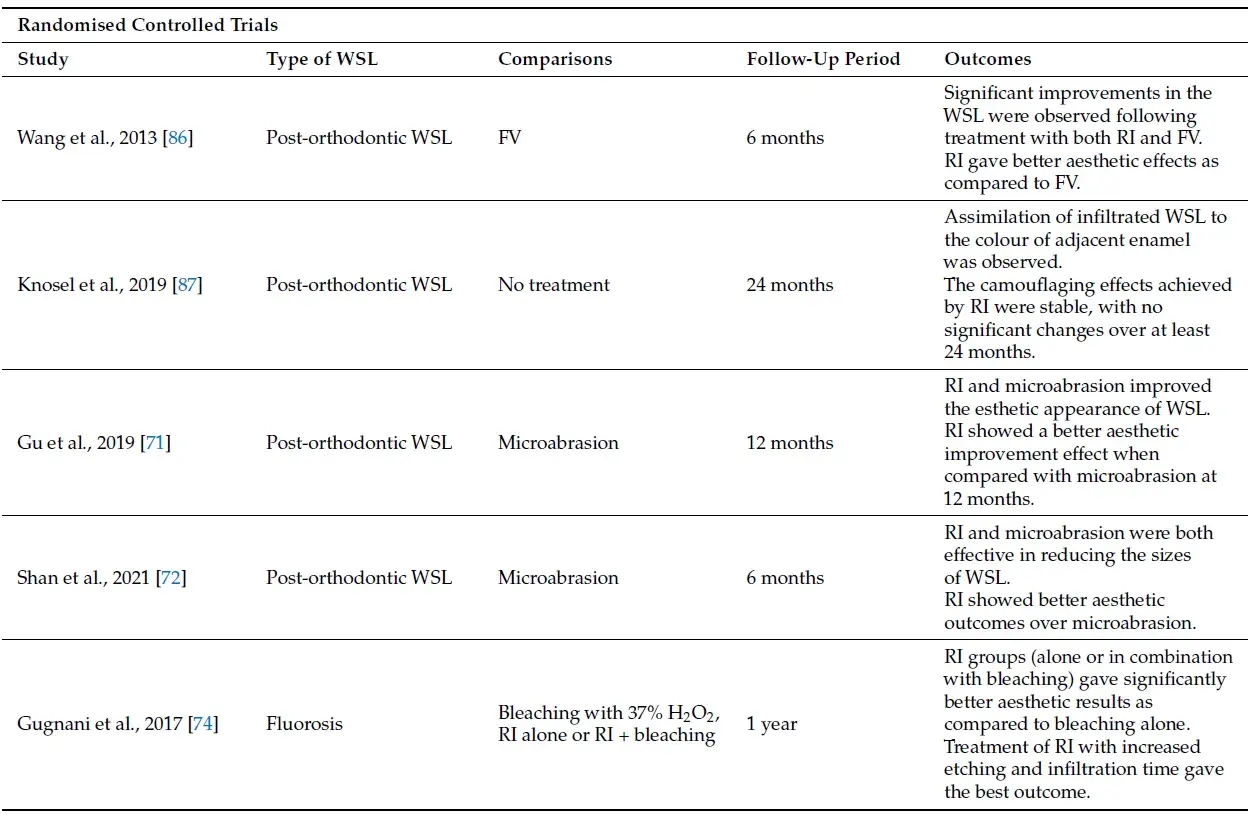
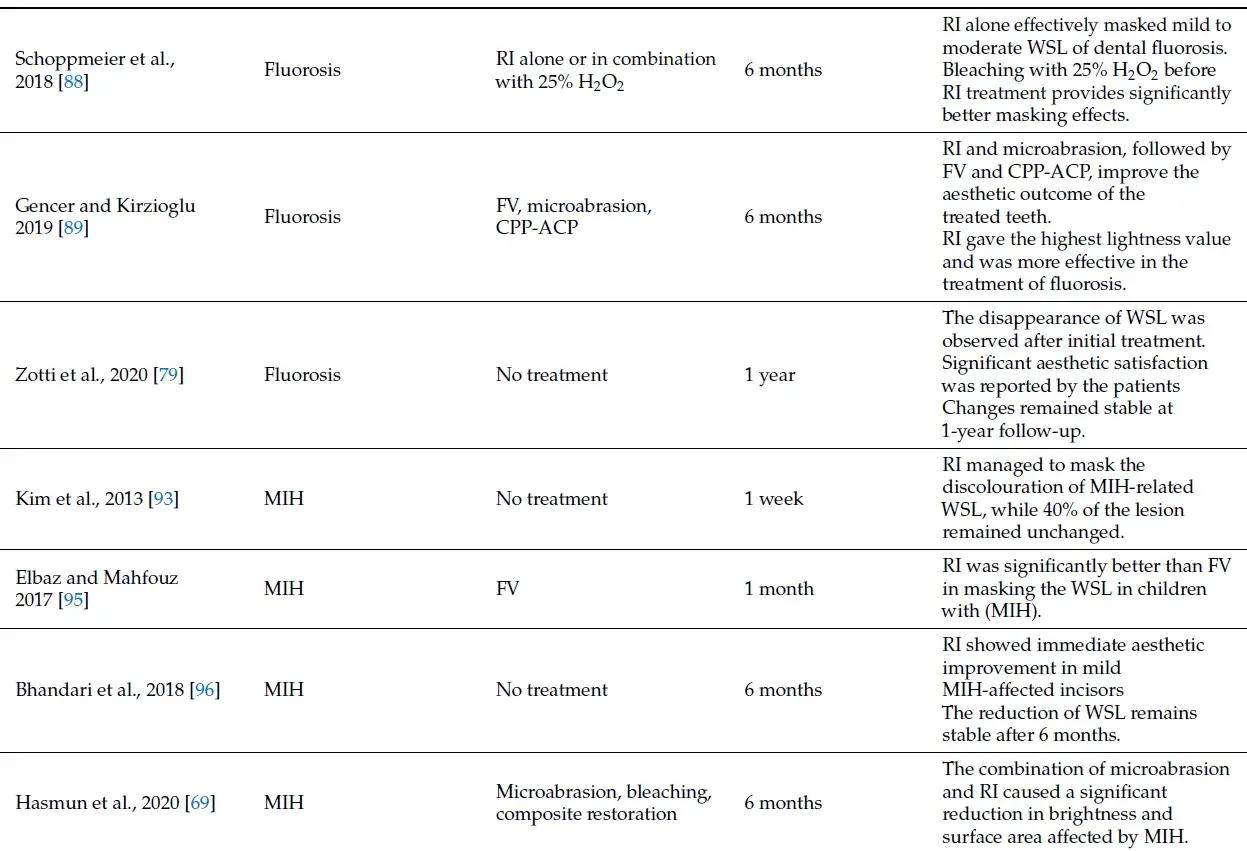
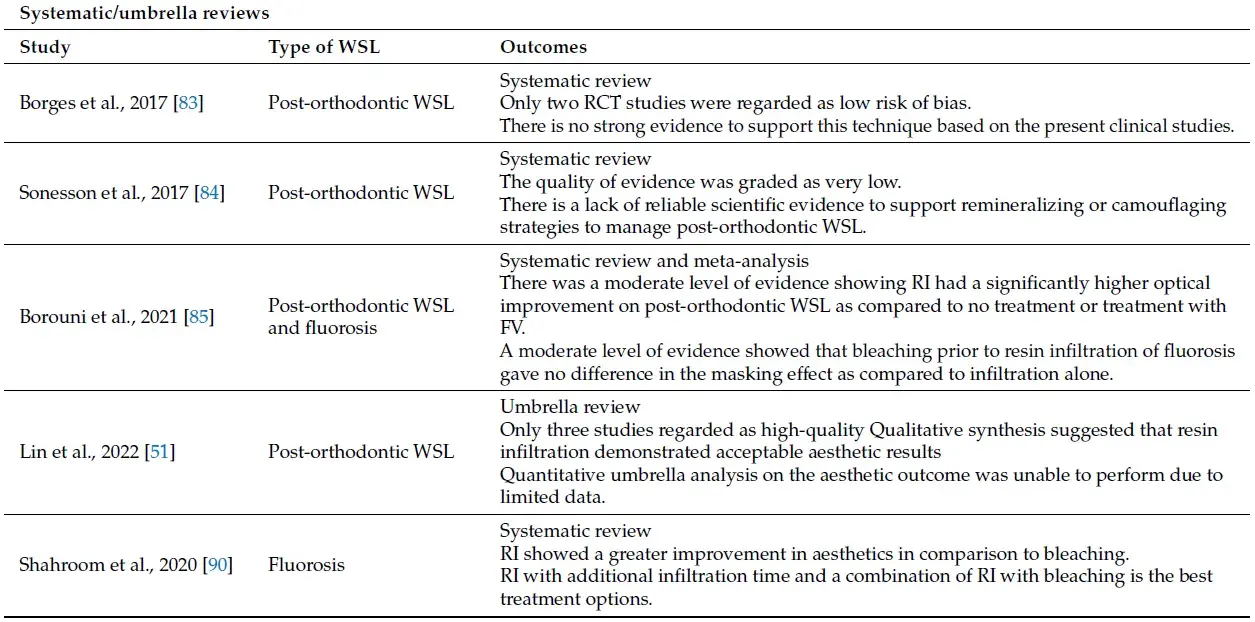
3.3. Limited Penetration Depth
One of the drawbacks of RI is the limited penetration depth of the material into the carious lesion. Despite various systematic reviews describing the efficacy of RI infiltrating the early enamel lesion, the penetration of the low-viscosity resin is limited to the enamel structure and did not perform well in the dentinal lesion. Peters et al. [45] reported that while the resin managed to infiltrate 100% into the inner enamel lesion (E2), only 64% of the outer dentine lesion (D1) was infiltrated by RI.
The histological difference between the enamel and dentinal carious lesion was thought to be the reason behind the poor penetration ability of the resin. The dentinal tubules, upon demineralisation, will be enlarged, creating a pathway for the outflow of the dentinal fluids into the lesion. This later prevents a complete penetration of the resin into the dentinal lesion [97].
Schneider et al. [98] studied the penetration ability of RI on extracted teeth using microcomputed tomography. They concluded that the resin was incapable of completely infiltrating the advanced carious lesion, with areas of resin inhomogeneity found within the lesion. Several in vitro studies on bovine and extracted premolar teeth reported the mean penetration depth of the RI to be less than 70% [99–101]. In different ICDAS codes, better penetration was reported in enamel lesions (ICDAS code 2–3) in contrast to the deeper lesion [102]. This was further supported by a systematic review and meta-analysis conducted by Liang et al. [103] and later Soveral et al. [82], where both authors described the limited ability of the resin to infiltrate deep into the dentinal lesion.
The penetration depth of RI in primary teeth is better as compared to the permanent teeth. Soviero et al. [104] reported a penetration depth between 70 to 80% in deciduous molars viewed under the scanning electron microscope, while although not significant, Aswani et al. [105] also observed higher penetration ability of the material into the carious lesion of primary teeth. The difference is attributed to the difference in the enamel structure between the two types of dentition, where deciduous enamel is less mineralised and thinner, providing a better platform for the resin to infiltrate the lesion faster and deeper.
The limited penetration depth of the resin will have some clinical implications. Liang et al. [103] suggested that the clinician should appropriately select which of the non-cavitated carious lesion is suitable for RI, guided by the depth of the lesion. This is also true for deeper WSL found on incisors teeth diagnosed with MIH. Due to the variety in the lesion depth of MIH-affected incisors, the masking ability of the RI might differ between cases; thus, case selection is very important to ensure an optimum aesthetic result.
3.4. Not Radiopaque
Ideally, a dental material should possess a certain degree of opacity in the radiograph. It is of paramount importance for the clinician to be able to distinguish the material against the surrounding background of enamel and dentine. This allows the clinician to assess the quality and clinical performance of the material. Apart from that, the presence of secondary caries, microleakage, marginal adaptation and overhangs can be detected clearly in the radiograph [106]. International Standard Organisation (ISO) recommends a dental material should have a radiopacity equivalent to or greater than the radiopacity produced by the thickness of pure aluminium [107].
However, this is not the case with RI. The RI is radiolucent in the radiograph, showing no clear distinction between the material and the infiltrated enamel and dentinal lesion. This will cause problems for the clinician as the penetration depth of the resin would not be visible radiographically. Further, the potential caries progression of the infiltrated lesion could not be monitored. Instead, multiple radiographs need to be taken periodically, and comparisons between radiographs need to be made for the sake of monitoring the success or failure of the infiltration technique. In addition, medico-legal issues, such as a dispute over the treatment charges, may arise. However, the clinician would face difficulty in proving that the carious lesion has been infiltrated with RI due to the radiolucency of the material [108].
This ‘loophole’ is worth to be explored. Thus, it is not surprising that the development of RI with radiopaque properties has been conducted actively by some researchers, which will be discussed in the next section.
3.5. Long-Term Colour and Material Stability
Aesthetic demand by parents and teenagers has increased over the years. The demands are even escalated when there are discrepancies in terms of colour, shape and alignment involving their teeth. With regards to restoring the anterior teeth, the colour stability of the resin-based material is one of the components that determine the long-term success of the material [109–111].
RI has been associated with poor long-term colour stability [109]. The infiltrated teeth are unable to retain the masking effects and are susceptible to staining by the colouring agents present in the diet, as proved by various in vitro studies. Significant colour changes have been reported following immersion with coffee [110,111], tea [110], red wine [112,113] and grape juice [114]. The colour changes were also more apparent in resin-infiltrated teeth as compared to teeth treated with remineralising agents [115]. The staining effects may be influenced by the duration of exposure and the intensity of the colouring [109].
The composition and ‘behaviour’ of the resin have been identified as one of the factors that contribute to the problem. TEDGMA has been proven to have the highest penetration coefficient as compared to others. However, TEDGMA also shows a high degree of water absorption owing to the presence of ether bonds which are said to be hydrophilic in nature [116]. In addition, the resins present with low filler content, which subjects the material to a certain degree of polymerisation shrinkage. The coefficient of thermal expansion also differs between the enamel structure and the resin. Over time, this unsynchronised expansion can lead to the formation of microcracks. Microcracks and material shrinkage increased the tendency of the material to absorb water as the material continued to degrade over time. This leads to discolouration of the material and subsequently affects the aesthetic outcome of the treated teeth [110].
Surface roughness also has been implicated as the cause of staining, mainly involving resin-based restoration [112]. The rough surface can act as the niche for dental plaque and bacterial adhesion, including stains and colouring from the diet. The minimal limit for acceptable surface roughness is valued at around 200 nm. Beyond this, plaque accumulation is inevitable [117]. Under vicious cycles of pH alteration, the bacterial and plaque accumulation subsequently cause degradation of the resin material, demineralisation of the enamel and potential formation of secondary caries [118]. Changes in surface integrity increase the tendency of the infiltrated teeth towards water absorption, which leads to surface staining and loss of surface gloss [119]. This has been proven by a number of in vitro studies conducted using bovine and extracted human teeth. Most of the authors were in agreement as they observed increased surface roughness and higher discolouration of the infiltrated teeth, even after polishing, as compared to sound enamel structure [110,118,119].
Despite the long-term colour instability, repolishing the surface of infiltrated teeth has improved the brightness of the discoloured surface [112,120]. Paris et al. [78] suggested that clinicians should wipe off the excess resin from the surface before light-curing to prevent excess material from sticking on the enamel surface, potentially attracting plaque accumulation. Further, the same authors also advocate polishing the surface post-infiltration. In addition, bleaching of the infiltrated surface has been suggested to improve discolouration [116,121]. The latter author also stated that surface bleaching did not interfere with the caries-arrest ability of RI. However, the use of bleaching agents among the paediatric population should be treated with caution to prevent unwanted iatrogenic damage to the gingiva and tooth structure, as described earlier.
Interestingly, clinical studies of resin-infiltrated anterior teeth did not report any significant discolouration or staining effects post-infiltration. Nevertheless, most of the clinical studies were of short-term follow-up, with the longest follow-up period reported by Knosel et al. [87] at 24 months. Meyer-Lueckel et al. [122] and Paris et al. [46] reported a longer follow-up period of four and seven years, respectively, for the infiltrated lesion on the proximal surface. However, colour changes were not part of their assessment, most probably due to the lesion located in the non-aesthetic zone. Further, the patient is brushing efficiency and frequency of dietary intake, in this case, those that can cause surface discolouration, such as coffee as well as a smoking habit, may play a role with regards to the staining tendency of the infiltrated surface. Thus, a true reflection on the colour stability of the resin-infiltrated anterior teeth could not be made. Despite that, the clinician should warn the parents and patient regarding the potential staining effects of the resin-infiltrated teeth and advice them for regular polishing and avoidance of certain foods and beverages that could stain the tooth structure if possible. Educating the parents and patients is the key element in paediatric dentistry, which helps the clinician in managing their expectations and avoidance of future disputes over the treatment provided.
4. Future: Innovation to Improve the Current RI
4.1. RI with Remineralising and Antibacterial Properties
Incorporation of an antibacterial or remineralising agent into the current RI formulation will offer huge advantages to the patients. While the former prevents bacterial colonisation at the infiltrated enamel surface, the latter enhances the remineralisation of the lesion and improves the hardness and resistance of the lesion toward a future acidic challenge [123]. With regards to Paediatric Dentistry, this is highly desirable and advantageous, especially in high-caries risk children where the progression of the lesion can be halted immediately, and more focus can be shifted to improving the child’s oral care habits and other preventive measures.
Bioactive glass (BAG), hydroxyapatite particles (HAP) and calcium phosphate (CaP) in the form of fillers are among the remineralising agents that have been incorporated in various dental restorative materials with great success. Apart from its anticaries activities, it also reduces the polymerisation shrinkage of the resin-based material. Similar concepts and ideas can be adopted in the formation of a RI with remineralising and antibacterial properties. One problem that might arise following the incorporation of fillers within the RI monomer matrix is the increase in viscosity and stiffness of the material, which will affect the penetration ability of the resin. It was suggested that the concentration of filler within a resin-based dental material should not exceed 15% of the total weight ratio [124]. However, with the advancement in the field of nanotechnology, a nano-sized filler can be formulated. This will allows the incorporation of the remineralising and antibacterial agent into the RI monomer matrix without affecting its penetrative ability.
Hashemian et al. [125] investigated the penetration ability, mechanical properties and calcium release ability of their experimental RI consisting of 10% polyurethane acrylate oligomer (PUA) and 88% TEDGMA. The resin showed a good penetration coefficient of 133 cm/s, exceeding the minimum threshold of 100 cm/s required for effective penetration of resin into the enamel porosity. The penetration depth was up to 120 m. The authors later added either HAP or BAG as filler into the formulation of their experimental resin. The incorporation of both fillers increased the microhardness and modulus of elasticity of the RI, as well as water sorption and solubility of the material. The latter outcome might reduce the colour stability of the material, although the degree of conversion (DC) did not significantly differ from the commercial RI. The DC value here is closely related to the water sorption and solubility of the material, where low DC is correlated with high water sorption and solubility, which will contribute to the poor clinical performance of the material [126]. Further, the author reported that the addition of HAP is more homogenous but releases fewer calcium ions which contribute to lower demineralisation resistance as compared to BAG-added RI.
A similar outcome was observed by Prodan et al. [127] using the fluoride-releasing glass filler, where incorporation of the filler improves the DC and strength of the material and inhibits demineralisation through the fluoride-releasing effect. However, contrasting findings were reported by Sfalcin et al. [128] in terms of water sorption and solubility of their experimental resin containing 25% ethoxylated bisphenol A dimethacrylate (BisEMA) and 75% TEDGMA doped with either HAP and BAG. In their study, the addition of either HAP and BAG produced lower water sorption and solubility and significantly higher DC value, microhardness and modulus of elasticity as compared to the commercial RI. This promising outcome proved that the use of HAP and BAG as fillers in the RI system carries the potential to improve the mechanical properties and caries arrest ability of the existing RI.
The use of CaP as fillers in RI was the subject of investigations conducted by Sfalcin et al. [128], Dai et al. [129] and Obeid et al. [130]. Sfalcin [128] and Dai [129] incorporated the amorphous CaP particles into their respective experimental RI monomer by the weight ratio of 10% and between 10 to 30%, respectively, while Obeid et al. [130] used nanofibers of polylactic acid (PLA) filled with CaP incorporated into silica network (PLA-SiO2-CaP) as their filler material. The addition of CaP increased the surface hardness and the DC value of the RI, and positive calcium and phosphate ion release were observed under pH-cycling challenge for PLA-SiO2-CaP and RI-30% CaP, respectively [129,130]. The deposition of interprismatic mineral crystal was observed by Dai et al. [129], suggesting the remineralising potential of the material. Interestingly, the addition of CaP increased the water sorption and solubility of the RI as compared to HAP- and BAG-added RI, respectively [128].
Apart from that, the effects of the incorporation of antibacterial agents as fillers on the mechanical properties and caries arrest ability of the RI also have been reported in the literature [97,131–136]. Kielbassa et al. [97] investigated the effectiveness and penetration ability of silver-enhanced RI. Silver, which is well-known for its antibacterial effects, was incorporated into the RI in the form of nanosilver particles (AgNP). Using the innovative tunnelling approach, the use of the experimental resin as an external infiltrant penetrated the proximal dentinal lesion and occluded the pores, similar to the commercial RI, suggesting that the addition of silver did not interfere with the penetration ability of the material. Further, the presence of silver may enhance the antibacterial effects of the material.
Another innovative antibacterial infiltrant was described by Cuppini et al. [135]. They used the ionic liquid 1-n-butyl-3-methylimidazolium bis(trifluoromethanesulfonyl)imide (BMI.NTf2) encapsulated with methacrylate-based microcapsules as the RI fillers. BMI.NTf2 was previously used as an antibacterial coating on polymers and implant surfaces [137], showing good antibacterial effects against S. mutans [138]. The addition of the fillers did not alter the handling and mechanical behaviour of the material, represented by the similar tensile strength of the commercial and experimental RIs. Further, the addition of 5% and 10% by weight ratio of the fillers reduced the surface free energy (SFE) and increased the contact angle of the material. This subsequently increases the hydrophobicity and reduces the wettability of the resin, improving its hydrolytic stability in the oral environment. This feature also has antibiofilm effects, as increased contact angle and low SFE could impair bacterial adherence and colonisation [139]. However, the penetration ability and other mechanical properties of the ionic liquid-loaded RI were not assessed by the authors.
The use of chlorhexidine diacetate salts [132], iodoniumsalts [133], quaternary ammoniumbased resinmonomer [134] and guanidine hydrochloride [140] were also being investigated as potential antibacterial RI fillers. The addition of chlorhexidine and iodonium salts improved the DC andmicrohardness of the experimental resin and inhibited the S. mutans and L. acidophilus biofilm, while the incorporation of the quaternary ammonium-based resinmonomer at 2.5% to 10% by weight ratio reduced the S. mutans bacterial count without affecting the surface roughness of thematerial. In addition, the incorporation of guanidine hydrochloride as filler of RI was also effective in disrupting the growth of S. mutans biofilm without affecting the SFE, DC and contact angle of the material. On the other hand, Skuca-Nowak et al. [131] and Fisher et al. [136] used metronidazole, an antibiotic that is highly effective against anaerobic bacteria and protozoa mixed with a methacrylate monomer (PMMAn) as the RI filler. The experimental resinmanaged to penetrate the demineralised enamel lesion up to 40 maswell as the demineralised root cementum and dentine. However, the relevance of using metronidazole might be questioned as the antibiotic is less effective against cariogenic bacteria.
Conclusively, the addition of remineralising and antibacterial agents as the nano-sized filler has opened a new prospective in the development of a RI with enhanced anticaries properties. Although most of the studies were in vitro based, and some did not evaluate the penetration ability of the material on enamel lesion, the potential of those materials are massive and promising. The path has been laid, and with further research and clinical trials, it is just a matter of time before the products based on this concept hit the market.
4.2. Self-Etching RI
The whole process of infiltrating a non-cavitated carious lesion involves at least five major steps, which include the isolation, application of acid etching, ethanol and dualapplication of RI with a 40-s light curing procedure in between them. The commercial RI uses 15% hydrochloric acid as surface pre-treatment before infiltrating the carious lesion with resin. This will sufficiently erode the surface layer and allows the penetration of the resinous material into the body lesion. The idea of developing a self-etching RI is well appreciated, especially in the field of Paediatric Dentistry. This would cut down the lengthy procedure, providing better management of the paediatric patient, especially those with short-term attention spans [141].
Wang et al. [142] have investigated the penetrative ability of their experimental self-etching RI, which consists of the resin monomer TEDGMA and phosphoric acid 2-hydroxyethyl methacrylate ester (PAM). Using the artificial WSL on bovine teeth, the composition of 75% TEDGMA: 25% PAM showed some potential as the penetration depth was found to be similar to the control when no acid etching was performed prior to the infiltration procedure. The experimental resin also demonstrated acceptable viscosity, which allows the material to penetrate the lesion.
The author stated that there is an inverse relationship between the ratio of PAM and the pH of the material, whereas, as the ratio of PAM increases, the pH becomes more acidic, but the material becomes more stiff and viscous. This will reduce the penetration ability of the resin and prevents it from infiltrating deeper lesion. Thus, they suggested the ratio of 75% TEDGMA: 25% PAM as the best ratio to be used as a self-etching RI.
Nevertheless, this product is still in its preliminary stages, and further research has been recommended by the author to explore its potential in infiltrating the WSL under different experimental settings.
4.3. Radiopaque RI
As mentioned in the previous section, the radiolucent appearance of the infiltrated lesion in the radiograph causes difficulty for the clinician to monitor the success or progress of the lesion. Therefore, the development of a RI with radiopaque properties is highly desirable. Moeinian [108] investigated the use of strontium hydroxyapatite (SrHA) nanoparticles and strontium bioglass (SrBG) as a filler mixed into the resin monomer TEDGMA. The author found out that the mixture resulted in a below-average radiopacity and increased the viscosity of the RI, thus limiting its potential to infiltrate the carious lesion.
The author later used barium and tin methacrylate monomers as potential radiopaque material. The incorporation of both radiopaque resin monomers produced a homogenous mixture with TEDGMA with low viscosity and contact angle values. The experimental RIs were later used to infiltrate artificially-created WSL. Although both mixtures managed to infiltrate the lesion to a certain depth, they were not clearly visible in the digital radiograph. The author concluded that despite meeting the required standard criteria of radiopacity, both experimental resins produced radiopacity lower than enamel, making it undiscernible in the radiograph. Increasing the volume of the radiopaque resin monomer is not possible as it would impair the mechanical properties of the RI [108].
Efforts in formulating a radiopaque RI were also made by Pedreira et al. [143] and Nowak-Wachol et al. [144]. The former authors investigated the radiopacity potential of an experimental RI, using either barium or zirconium oxide particles which served as a filler and incorporated into the RI monomer. In addition, the water sorption, solubility and penetration depth of the experimental resin were also evaluated. Their in vitro study showed that the mixture of 45% zirconium oxide particles (by weight) with RI monomer produced radiopacity higher than enamel when viewed using a digital radiograph. Further, this experimental resin demonstrated a comparable penetration depth but lower water sorption and solubility when compared to the commercial RI, suggesting its promising potential in dentistry. On the other hand, the mixture of 25% barium oxide particles (by weight) with RI monomer did not perform well for all the investigated outcomes [143].
Nowak-Wachol et al. [144], on the other hand, used yttrium trifluoride (YF3) incorporated in their experimental RI consisting of resin monomer TEDGMA, HEMA and a bioactive methacrylate monomer, PMMAn with build-in metronidazole. They hypothesised the potential antibacterial and radiopaque effects of the experimental RI following the addition of YF3. However, the penetration of the experimental resin into the carious root dentine was hampered by the inability of YF3 to penetrate deeper into the lesion due to its large particle size.
The incorporation of 45% (by weight) zirconium oxide showed some potential and is by far the best option available at the moment. It truly has shed some light on the efforts of producing a radiopaque RI. Nevertheless, since it was conducted on an in vitro basis, whether the zirconium-enhanced RI would perform well in the in vivo or ex vivo setting is still under investigation. Further research is still needed to prove the potential of this material.
4.4. Micro-Filled RI
The current RI is highly effective in arresting the non-cavitated enamel lesion by occluding the pores and blocking the diffusion pathways of the minerals and acids. However, as pointed out before, the penetration ability of the RI is limited to the enamel lesion only with the ICDAS score of 2 and 3 [96], and the performance in arresting deep dentinal carious lesions is poor, with less than 65% of the outer dentinal lesion (D1) were completely infiltrated [45]. This failure is attributed to no filler being incorporated within the RI matrix monomer, contributing to high polymerisation shrinkage and lack of ‘bulk’ of the material.
This has led Meyer-Lueckel and Paris [145,146], together with their innovative research team members, to conduct a series of experimental studies investigating the possibility of incorporating fillers into the RI monomer matrix without jeopardising its penetrative ability. In their experimental study, two organic fillers of different particle sizes and one glass filler at 0.7 m were mixed with the RI monomer TEDGMA, reaching up to 55% of filler content. The percentage of lesion penetration for each formulation was assessed using the artificial enamel caries model with confocal laser scanning microscopy. The outcome showed that the filler size influenced the penetration ability of the resins, where RI with 42 m of organic filler displayed an indifferent penetration percentage as the commercial unfilled RI [145]. The success of this early experiment has paved the way for the improvisation of the current RI formulation and indirectly improved the mechanical properties of the material as well.
Subsequently, using a similar formulation, the micro-filled RI was applied to the natural enamel carious lesion of extracted teeth with ICDAS scores of 2, 3 and 5. The latter score indicates a cavitated carious lesion that has extended into the dentine, with minimal loss of enamel structure. The micro-filled RI showed similar efficacy in penetrating the natural enamel carious lesion as the commercial unfilled resin but managed to fill the ICDAS 5 cavity as assessed through the dual-fluorescence staining and confocal microscopy [146].
The presence of the organic filler has given more ‘bulk’ to the RI, contributing to the success of the newly formulated material in filling up the small cavity with less polymerisation shrinkage. However, the authors did not assess the compressive strength of the micro-filled RI and compared it with the conventional resin composite or glass ionomer restoration. It is also interesting to see whether this micro-filled RI can be used as a fissure sealant and restoration of small abrasion cavities. If proven, this certainly will add more versatility to the material, supporting the micro-invasive technique in the management of carious enamel lesions in children. Further, the performance of the material in a clinical setting is yet to be determined and warrants more clinical trials in the future. Despite that, the future of RI in Paediatric Dentistry is definitely bright and promising.
A summary of the main laboratory-based studies conducted to improve the current RI is shown in Table 4.
Table 4. Summary of the main laboratory-based studies conducted to improve the current RI.
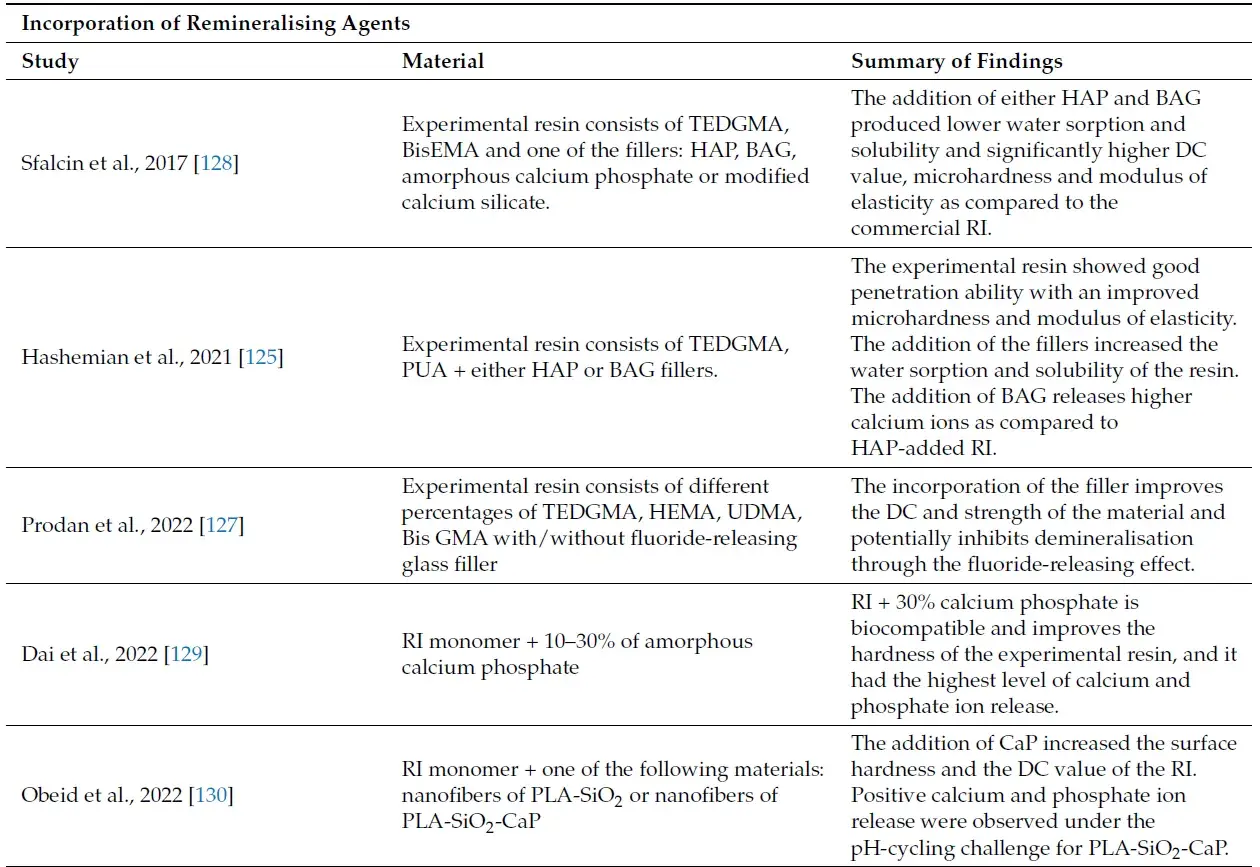
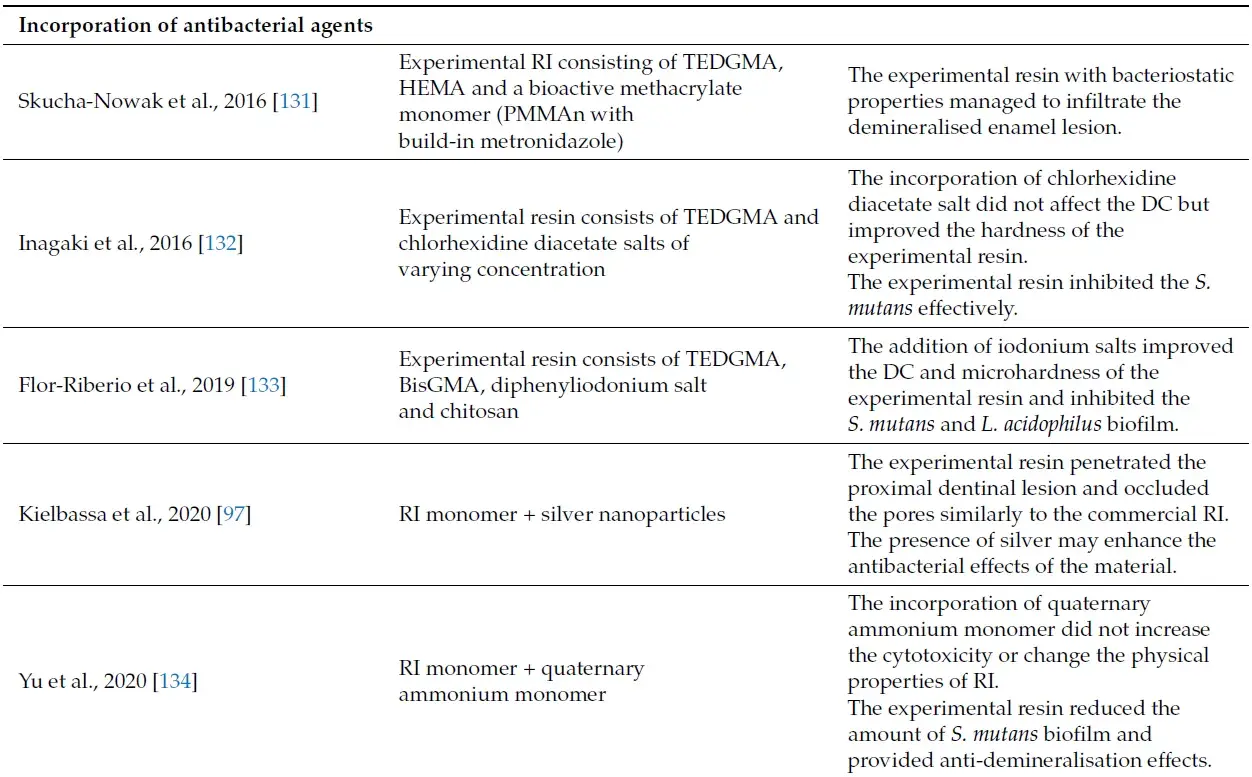
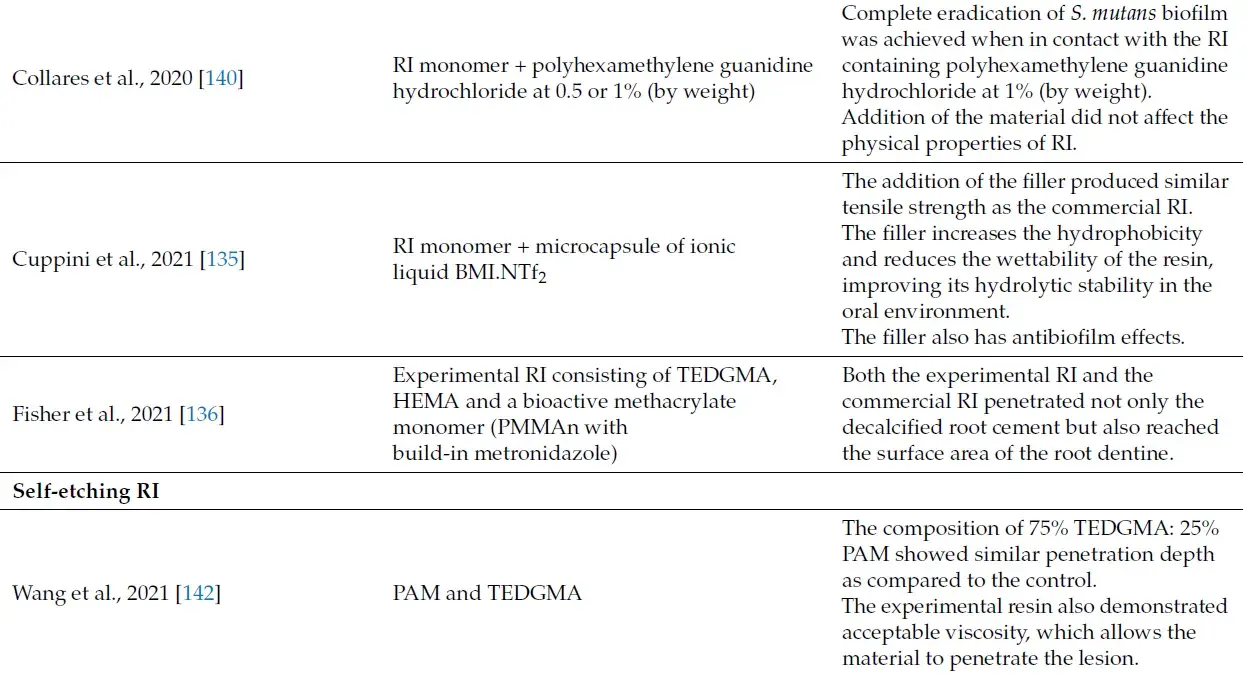
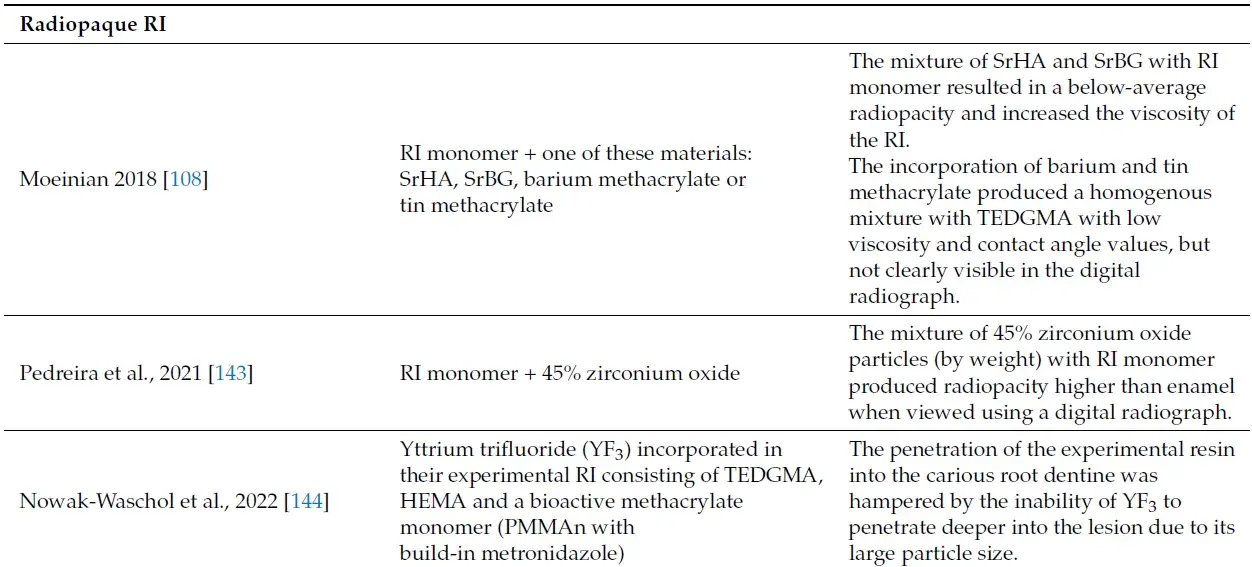
5. Conclusions
RI has emerged as one of the important micro-invasive techniques in addressing non-cavitated and white-spot enamel lesions in children and adolescents. However, some issues of concern have been raised over the radiopacity, colour stability and its usage in deep dentinal lesions. Improvisation of the current RI is ongoing actively at the moment, aiming to address the clinical issues associated with the material. Nevertheless, most of the studies are laboratory-based, which might lead to different outcomes clinically. Thus, further research in terms of clinical trials is needed.
Limitations
This narrative review was conducted by screening through all the relevant studies in the literature to support the ideas and arguments that we have pointed out in this review. This includes umbrella and systematic reviews, randomised clinical trials, non-randomised clinical studies and laboratory-based studies. The level of bias and strength of the studies were not assessed. As discussed before, although the value of laboratory-based studies cannot be denied, their impacts and effects in the actual clinical setting might be different.
List of authors
Nabihah Dziaruddin
Ahmad Shuhud Irfani Zakaria
References
1. Kassebaum, N.J.; Bernabé, E.; Dahiya, M.; Bhandari, B.; Murray, C.J.; Marcenes,W. Global burden of untreated caries: A systematic review and metaregression. J. Dent. Res. 2015, 94, 650–658. [CrossRef]
2. Velasco, S.R.M.; Pistelli, G.C.; Razera, F.P.M.; Menezes-Silva, R.; Bastos, R.S.; Navarro, M.F.L. Dental caries spectrum profile in Brazilian public school children and adolescents. Braz. Oral Res. 2021, 35, e067. [CrossRef] [PubMed]
3. Innes, N.P.T.; Chu, C.H.; Fontana, M.; Lo, E.C.M.; Thomson, W.M.; Uribe, S.; Heiland, M.; Jepsen, S.; Schwendicke, F. A Century of Change towards Prevention and Minimal Intervention in Cariology. J. Dent. Res. 2019, 98, 611–617. [CrossRef]
4. Frencken, J.E.; Peters, M.C.; Manton, D.J.; Leal, S.C.; Gordan, V.V.; Eden, E. Minimal intervention dentistry for managing dental caries-a review: Report of a FDI task group. Int. Dent. J. 2012, 62, 223–243. [CrossRef] [PubMed]
5. Buonocore, M.G. A simple method of increasing the adhesion of acrylic filling materials to enamel surfaces. J. Dent. Res. 1955, 34, 849–853. [CrossRef] [PubMed]
6. Bowen, R.L. Properties of a silica-reinforced polymer for dental restorations. J. Am. Dent. Assoc. 1963, 66, 57–64. [CrossRef] [PubMed]
7. Giray, F.E.; Durhan, M.A.; Haznedaroglu, E.; Durmus, B.; Kalyoncu, I.O.; Tanboga, I. Resin infiltration technique and fluoride varnish on white spot lesions in children: Preliminary findings of a randomized clinical trial. Niger. J. Clin. Pract. 2018, 21, 1564–1569. [PubMed]
8. Davila, J.M.; Buonocore, M.; Greeley, C.B.; Provenza, V. Adhesive penetration in human artificial and natural white spots. J. Dent. Res. 1975, 54, 999–1008.
9. Robinson, C.; Hallsworth, A.S.;Weatherell, J.A.; Kunzel, W. Arrest and control of carious lesions: A study based on preliminary experiments with resorcinol-formaldehyde resin. J. Dent. Res. 1976, 55, 812–855. [CrossRef]
10. Kantovitz, K.R.; Pascon, F.M.; Nobres-dos-Santos, M.; Puppin-Rontani, R.M. Review of the effects of infiltrants and sealers on non-cavitated enamel lesions. Oral Health Prev. Dent. 2010, 8, 295–305.
11. Robinson, C.; Brookes, S.J.; Kirkham, J.; Wood, S.R.; Shore, R.C. In vitro studies of the penetration of adhesive resins into artificial caries-like lesions. Caries Res. 2001, 5, 136–141. [CrossRef] [PubMed]
12. Meyer-Lueckel, H.; Mueller, J.; Paris, S.; Hummel, M.; Kielbassa, A.M. The penetration of various adhesives into early enamel lesions in vitro. Schweiz. Monatsschr. Zahnmed. 2005, 115, 316–323. [PubMed]
13. Mueller, J.; Meyer-Lueckel, H.; Paris, S.; Hopfenmuller, W.; Kielbassa, A.M. Inhibition of lesion progression by the penetration of resins in vitro: Influence of the application procedure. Oper. Dent. 2006, 31, 338–345. [CrossRef] [PubMed]
14. Gomez, S.S.; Basili, C.P.; Emilson, C.G. A 2-year clinical evaluation of sealed noncavitated approximal posterior carious lesions in adolescents. Clin. Oral Investig. 2005, 9, 239–243. [CrossRef] [PubMed]
15. Martignon, S.; Ekstrand, K.R.; Ellwood, R. Efficacy of sealing proximal early active lesion: An 18-months clinical study evaluated by conventional and subtraction radiography. Caries Res. 2006, 40, 382–388. [CrossRef]
16. Meyer-Lueckel, H.; Paris, S.; Mueller, J.; Cölfen, H.; Kielbassa, A.M. Influence of the application time on the penetration of different dental adhesives and a fissure sealant into artificial subsurface lesions in bovine enamel. Dent. Mater. 2006, 22, 22–28. [CrossRef]
17. Paris, S.; Meyer-Lueckel, H.; Cölfen, H.; Kielbassa, A.M. Resin infiltration of artificial enamel caries lesions with experimental light curing resins. Dent. Mater. J. 2007, 26, 582–588. [CrossRef]
18. Paris, S.; Meyer-Lueckel, H.; Cölfen, H.; Kielbassa, A.M. Penetration coefficients of commercially available and experimental composites intended to infiltrate enamel carious lesions. Dent. Mater. 2007, 23, 742–748. [CrossRef] [PubMed]
19. Buckton, G. Interfacial Phenomena in Drug Delivery and Targeting, 1st ed.; Harwood Academic Publishers: Chur, Switzerland, 1995; pp. 28–33.
20. Fan, P.L.; Seluk, L.W.; O’Brien,W.J. Penetrativity of sealants. J. Dent. Res. 1975, 54, 262–264.
21. Meyer-Lueckel, H.; Paris, S. Progression of artificial enamel caries lesions after infiltration with experimental light curing resins. Caries Res. 2008, 42, 117–124. [CrossRef]
22. Meyer-Lueckel, H.; Paris, S. Improved resin infiltration of natural caries lesions. J. Dent. Res. 2008, 87, 1112–1116. [CrossRef] [PubMed]
23. Meyer-Lueckel, H.; Paris, S. Infiltration of natural caries lesions with experimental resins differing in penetration coefficients and ethanol addition. Caries Res. 2010, 44, 408–414. [CrossRef] [PubMed]
24. Paris, S.; Meyer-Lueckel, H. Inhibition of caries progression by resin infiltration in situ. Caries Res. 2010, 44, 47–54. [CrossRef] [PubMed]
25. Meyer-Lueckel, H.; Chatzidakis, A.; Naumann, M.; Dörfer, C.E.; Paris, S. Influence of application time on penetration of an infiltrant into natural enamel caries. J. Dent. 2011, 39, 465–469. [CrossRef] [PubMed]
26. Hicks, M.J.; Silverstone, L.M. Internal morphology of surface zones from acid-etched caries-like lesions: A scanning electron microscopic study. J. Dent. Res. 1985, 64, 1296–1301. [CrossRef]
27. Buonocore, M.G.; Matsui, A.; Gwinnett, A.J. Penetration of resin dental materials into enamel surfaces with reference to bonding. Arch. Oral Biol. 1968, 13, 61–70. [CrossRef]
28. Gwinnett, A.J. Histologic changes in human enamel following treatment with acidic adhesive conditioning agents. Arch. Oral Biol. 1971, 16, 731–738. [CrossRef]
29. Gray, G.B.; Shellis, P. Infiltration of resin into white spot caries-like lesions of enamel: An in vitro study. Eur. J. Prosthodont. Restor. Dent. 2002, 10, 27–32.
30. Meyer-Lueckel, H.; Paris, S.; Kielbassa, A.M. Surface layer erosion of natural caries lesions with phosphoric and hydrochloric acid gels in preparation for resin infiltration. Caries Res. 2007, 41, 223–230. [CrossRef]
31. Paris, S.; Meyer-Lueckel, H.; Kielbassa, A.M. Resin infiltration of natural caries lesions. J. Dent. Res. 2007, 86, 662–666. [CrossRef]
32. Croll, T.P. Bonded resin sealant for smooth surface enamel defects: New concepts in ‘microrestorative’ dentistry. Quintessence Int. 1987, 18, 5–10. [PubMed]
33. Paris, S.; Soviero, V.M.; Chatzidakis, A.J.; Meyer-Lueckel, H. Penetration of experimental infiltrants with different penetration coefficients and ethanol addition into natural caries lesions in primary molars. Caries Res 2012, 46, 113–117. [CrossRef] [PubMed]
34. Qvist, V. Longevity of Restorations–"The Death Spiral". In Dental Caries: The Disease and its Clinical Management, 3rd ed.; Fejerskov, O., Nyvad, B., Kidd, E., Eds.;Wiley-Blackwell: New York, NY, USA, 2015; pp. 443–455.
35. Marinho, V.C. Cochrane reviews of randomized trials of fluoride therapies for preventing dental caries. Eur. Arch. Paediatr. Dent. 2009, 10, 183–191. [CrossRef] [PubMed]
36. Cochrane, N.J.; Reynolds, E.C. Calcium phosphopeptides-mechanism of action and evidence for clinical efficacy. Adv. Dent. Res. 2012, 4, 41–47. [CrossRef] [PubMed]
37. Kidd, E.A.M.; van Amerongen, J.P. The Role of Operative Treatment. In Dental Caries: The Disease and its Clinical Management, 3rd ed.; Fejerskov, O., Nyvad, B., Kidd, E., Eds.;Wiley-Blackwell: New York, NY, USA, 2015; pp. 245–250.
38. Willmot, D.R. White lesions after orthodontic treatment: Does low fluoride make a difference? J. Orthod. 2004, 31, 235–242. [CrossRef] [PubMed]
39. Ardu, S.; Castioni, N.V.; Benbachir, N.; Krejci, I. Minimally invasive treatment of white spot enamel lesions. Quintessence Int. 2007, 38, 633–636. [PubMed]
40. Ekstrand, K.R.; Bakhshandeh, A.; Martignon, S. Treatment of proximal superficial caries lesions on primary molar teeth with resin infiltration and fluoride varnish versus fluoride varnish only: Efficacy after 1 year. Caries Res. 2010, 44, 41–46. [CrossRef]
41. Page, L.A.F.; Beckett, D.; Ahmadi, R.; Schwass, D.R.; de la Barra, S.L.; Moffat, S.M.; Meldrum, A.; Thomson,W.M. Resin Infiltration of Caries in Primary Molars in a Community Setting: 24-Month Randomized Controlled Trial Findings. JDR Clin. Trans. Res. 2017, 2, 287–294.
42. Ammari, M.M.; Jorge, R.C.; Souza, I.P.R.; Soviero, V.M. Efficacy of resin infiltration of proximal caries in primary molars: 1-year follow-up of a split-mouth randomized controlled clinical trial. Clin. Oral Investig. 2018, 22, 1355–1362. [CrossRef]
43. Urquhart, O.; Tampi, M.P.; Pilcher, L.; Slayton, R.L.; Araujo, M.W.B.; Fontana, M.; Guzmán-Armstrong, S.; Nascimento, M.M.; Nový, B.B.; Tinanoff, N.; et al. Nonrestorative Treatments for Caries: Systematic Review and Network Meta-analysis. J. Dent. Res. 2019, 98, 14–26. [CrossRef]
44. Meyer-Lueckel, H.; Bitter, K.; Paris, S. Randomized controlled clinical trial on proximal caries infiltration: Three-year follow-up. Caries Res. 2012, 46, 544–548. [CrossRef] [PubMed]
45. Peters, M.C.; Hopkins, A.R.; Zhu, L.; Yu, Q. Efficacy of Proximal Resin Infiltration on Caries Inhibition: Results from a 3-Year Randomized Controlled Clinical Trial. J. Dent. Res. 2019, 98, 1497–1502. [CrossRef]
46. Paris, S.; Bitter, K.; Krois, J.; Meyer-Lueckel, H. Seven-year-efficacy of proximal caries infiltration-Randomized clinical trial. J. Dent. 2020, 93, 103277. [CrossRef]
47. Bakhshandeh, A.; Ekstrand, K. Infiltration and sealing versus fluoride treatment of occlusal caries lesions in primary molar teeth. 2-3 years results. Int. J. Paediatr. Dent. 2015, 25, 43–50. [CrossRef] [PubMed]
48. Barakat, I.; El-Soud, M.G.A. Resin infiltrate versus compomer as pit and fissure sealants of primary teeth: A randomized controlled clinical trial. Egypt. Dent. J. 2021, 67, 1033–1041. [CrossRef]
49. Slayton, R.L.; Urquhart, O.; Araujo, M.W.B.; Fontana, M.; Guzmán-Armstrong, S.; Nascimento, M.M.; Nový, B.B.; Tinanoff, N.; Weyant, R.J.; Wolff, M.S.; et al. Evidence-based clinical practice guideline on nonrestorative treatments for carious lesions: A report from the American Dental Association. J. Am. Dent. Assoc. 2018, 149, 837–849. [CrossRef] [PubMed]
50. Faghihian, R.; Shirani, M.; Tarrahi, M.J.; Zakizade, M. Efficacy of the resin infiltration technique in preventing initial caries progression: A systematic review and meta-analysis. Pediatr. Dent. 2019, 41, 88–94.
51. Lin, G.S.S.; Chan, D.Z.K.; Lee, H.Y.; Low, T.T.; Laer, T.S.; Pillai, M.P.M.; Yew, Y.Q.; Wafa, S.W.W.S.S.T. Effectiveness of resin infiltration in caries inhibition and aesthetic appearance improvement of white-spot lesions: An umbrella review. J. Evid. Based Dent. Pract. 2022, 22, 101723. [CrossRef]
52. Paris, S.; Meyer-Lueckel, H. Masking of labial enamel white spot lesions by resin infiltration–a clinical report. Quintessence Int. 2009, 40, 713–718.
53. Ogaard, B.; Rolla, G.; Arends, J. Orthodontic appliances and enamel demineralization. Part 1. Lesion development. Am. J. Orthod. Dentofacial Orthop. 1988, 94, 68–73. [CrossRef]
54. Benson, P.; Javidi, H.; DiBiase, A. What is the value of orthodontic treatment? Br. Dent. J. 2015, 218, 185–190. [CrossRef] [PubMed]
55. Sundararaj, D.; Venkatachalapathy, S.; Tandon, A.; Pereira, A. Critical evaluation of incidence and prevalence of white spot lesions during fixed orthodontic appliance treatment: A meta-analysis. J. Int. Soc. Prev. Community Dent. 2015, 5, 433–439.
56. Lucchese, A.; Gherlone, E. Prevalence of white-spot lesions before and during orthodontic treatment with fixed appliances. Eur. J. Orthod. 2013, 35, 664–668. [CrossRef] [PubMed]
57. Kidd, E.A.M.; Fejerskov, O. What constitutes dental caries? Histopathology of carious enamel and dentine related to the action of cariogenic biofilms. J. Dent. Res. 2004, 83 (Suppl. S1), C35–C38. [CrossRef]
58. Roberts,W.E.; Mangum, J.E.; Schneider, P.M. Pathophysiology of Demineralization, Part II: Enamel White Spots, Cavitated Caries, and Bone Infection. Curr. Osteoporos. Rep. 2022, 20, 106–119. [CrossRef] [PubMed]
59. O’Reilly, M.M.; Featherstone, J.D. Demineralization and remineralization around orthodontic appliances: An in vivo study. Am. J. Orthod. Dentofacial Orthop. 1987, 92, 33–40. [CrossRef]
60. Den Besten, P.K. Effects of fluoride on protein secretion and removal during enamel development in the rat. J. Dent. Res. 1986, 65, 1272–1277. [CrossRef]
61. Den Besten, P.; Li,W. Chronic fluoride toxicity: Dental fluorosis. Monogr. Oral Sci. 2011, 22, 81–96.
62. Weerheijm, K.L. Molar incisor hypomineralisation (MIH). Eur. J. Paediatr. Dent. 2003, 4, 114–120.
63. Lygidakis, N.A.; Wong, F.; Jälevik, B.; Vierrou, A.M.; Alaluusua, S.; Espelid, I. Best Clinical Practice Guidance for clinicians dealing with children presenting with Molar-Incisor-Hypomineralisation (MIH): An EAPD Policy Document. Eur. Arch. Paediatr. Dent. 2010, 11, 75–81. [CrossRef]
64. Whatling, R.; Fearne, J.M. Molar incisor hypomineralization: A study of aetiological factors in a group of UK children. Int. J. Paediatr. Dent. 2008, 18, 155–162. [CrossRef] [PubMed]
65. Crombie, F.; Manton, D.; Kilpatrick, N. Aetiology of molar-incisor hypomineralization: A critical review. Int. J. Paediatr. Dent. 2009, 19, 73–83. [CrossRef] [PubMed]
66. Silva, M.J.; Scurrah, K.J.; Craig, J.M.; Manton, D.J.; Kilpatrick, N. Etiology of molar incisor hypomineralization-A systematic review. Community Dent. Oral Epidemiol 2016, 44, 342–353. [CrossRef] [PubMed]
67. Butera, A.; Maiorani, C.; Morandini, A.; Simonini, M.; Morittu, S.; Barbieri, S.; Bruni, A.; Sinesi, A.; Ricci, M.; Trombini, J.; et al. Assessment of genetical, pre, peri and post natal risk factors of deciduous molar hypomineralization (DMH), hypomineralized second primary molar (HSPM) and molar incisor hypomineralization (MIH): A narrative review. Children 2021, 8, 432. [CrossRef] [PubMed]
68. Lygidakis, N.A.; Garot, E.; Somani, C.; Taylor, G.D.; Rouas, P.;Wong, F.S.L. Best clinical practice guidance for clinicians dealing with children presenting with molar-incisor-hypomineralisation (MIH): An updated European Academy of Paediatric Dentistry policy document. Eur. Arch. Paediatr. Dent. 2022, 3, 3–21. [CrossRef] [PubMed]
69. Hasmun, N.; Vettore, M.V.; Lawson, J.A.; Elcock, C.; Zaitoun, H.; Rodd, H.D. Determinants of children’s oral health-related quality of life following aesthetic treatment of enamel opacities. J. Dent. 2020, 98, 103372. [CrossRef]
70. Hu, H.; Feng, C.; Jiang, Z.;Wang, L.; Shrestha, S.; Yan, J.; Shu, Y.; Ge, L.; Lai,W.; Hua, F.; et al. Effectiveness of remineralizing agents in the prevention and reversal of orthodontically induced white spot lesions: A systematic review and network meta-analysis. Clin. Oral Investig. 2020, 24, 4153–4167. [CrossRef]
71. Gu, X.; Yang, L.; Yang, D.; Gao, Y.; Duan, X.; Zhu, X.; Yuan, H.; Li, J. Esthetic improvements of postorthodontic white-spot lesions treated with resin infiltration and microabrasion: A split-mouth, randomized clinical trial. Angle Orthod. 2019, 89, 372–377. [CrossRef]
72. Shan, D.; He, Y.; Gao, M.; Liu, H.; Zhu, Y.; Liao, L.; Hadaegh, F.; Long, H.; Lai, W. A comparison of resin infiltration and microabrasion for postorthodontic white spot lesion. Am. J. Orthod. Dentofacial Orthop. 2021, 160, 516–522. [CrossRef]
73. Knösel, M.; Attin, R.; Becker, K.; Attin, T. External bleaching effect on the color and luminosity of inactive white-spot lesions after fixed orthodontic appliances. Angle Orthod. 2007, 77, 646–652. [CrossRef]
74. Gugnani, N.; Pandit, I.K.; Gupta, M.; Gugnani, S.; Soni, S.; Goyal, V. Comparative evaluation of esthetic changes in nonpitted fluorosis stains when treated with resin infiltration, in-office bleaching, and combination therapies. J. Esthet. Restor. Dent. 2017, 29, 317–324. [CrossRef] [PubMed]
75. Bailey, D.L.; Adams, G.G.; Tsao, C.E.; Hyslop, A.; Escobar, K.; Manton, D.J.; Reynolds, E.C.; Morgan, M.V. Regression of post-orthodontic lesions by a remineralizing cream. J. Dent. Res. 2009, 88, 1148–1453. [CrossRef]
76. Kelleher, M. The law is an ass: Legal and ethical issues surrounding the bleaching of young patients’ discoloured teeth. Fac. Dent. J. 2014, 5, 56–67. [CrossRef]
77. Griffiths, F.; Parekh, S. Is it time to reconsider the use of vital teeth bleaching in children and adolescents in Europe? Eur. Arch. Paediatr. Dent. 2021, 22, 759–763. [CrossRef] [PubMed]
78. Paris, S.; Schwendicke, F.; Keltsch, J.; Dorfer, C.; Meyer-Lueckel, H. Masking of white spot lesions by resin infiltration in vitro. J. Dent. 2013, 41, e28–e34. [CrossRef] [PubMed]
79. Zotti, F.; Albertini, L.; Tomizioli, N.; Capocasale, G.; Albanese, M. Resin infiltration in dental fluorosis treatment-1-year follow-up. Medicina 2020, 57, 22. [CrossRef]
80. Saxena, P.; Grewal, M.S.; Agarwal, P.; Kaur, G.; Verma, J.; Chhikara, V. Clinical efficacy of resin infiltration technique alone or in combination with micro abrasion and in-office bleaching in adults with mild-to-moderate fluorosis stains. J. Pharm. Bioallied. Sci. 2021, 13, S301–S305. [CrossRef]
81. Zakizade, M.; Davoudi, A.; Akhavan, A.; Shirban, F. Effect of resin infiltration technique on improving surface hardness of enamel lesions: A systematic review and meta-analysis. J. Evid. Based Dent. Pract. 2020, 20, 101405. [CrossRef]
82. Soveral, M.; Machado, V.; Botelho, J.; Mendes, J.J.; Manso, C. Effect of resin infiltration on enamel: A systematic review and meta-analysis. J. Funct. Biomater. 2021, 12, 48. [CrossRef]
83. Borges, A.B.; Caneppele, T.M.; Masterson, D.; Maia, L.C. Is resin infiltration an effective esthetic treatment for enamel development defects and white spot lesions? A systematic review. J. Dent. 2017, 56, 11–18. [CrossRef]
84. Sonesson, M.; Bergstrand, F.; Gizani, S.; Twetman, S. Management of post-orthodontic white spot lesions: An updated systematic review. Eur. J. Orthod. 2017, 39, 116–121. [CrossRef] [PubMed]
85. Bourouni, S.; Dritsas, K.; Kloukos, D.;Wierichs, R.J. Efficacy of resin infiltration to mask post-orthodontic or non-post-orthodontic white spot lesions or fluorosis-A systematic review and meta-analysis. Clin Oral Investig 2021, 25, 4711–4719. [CrossRef] [PubMed]
86. Wang, L.; Jian, J.; Lu, H.F. Efficiency of resin infiltration versus fluride varnish for treatment of post-orthodontic white spot lesions. Chin. J. Tissue Eng. Res. 2013, 17, 5303–5308.
87. Knosel, M.; Eckstein, A.; Helms, H.J. Long-term follow-up of camouflage effects following resin infiltration of post orthodontic white-spot lesions in vivo. Angle Orthod. 2019, 89, 33–39. [CrossRef]
88. Schoppmeier, C.M.; Derman, S.H.M.; Noack, M.J.;Wicht, M.J. Power bleaching enhances resin infiltration masking effect of dental fluorosis. A randomized clinical trial. J. Dent. 2018, 79, 77–84. [CrossRef]
89. Gencer, M.D.G.; Kirzioglu, Z. A comparison of the effectiveness of resin infiltration and microabrasion treatments applied to developmental enamel defects in color masking. Dent. Mater. J. 2019, 38, 295–302. [CrossRef]
90. Shahroom, N.S.B.; Mani, G.; Ramakrishnan, M. Interventions in management of dental fluorosis, an endemic disease: A systematic review. J. Family Med. Prim. Care 2019, 8, 3108–3113.
91. Lopes, L.B.; Machado, V.; Botelho, J.; Haubek, D. Molar-incisor hypomineralization: An umbrella review. Acta Odontol. Scand. 2021, 79, 359–369. [CrossRef]
92. Bulanda, S.; Ilczuk-Rypuła, D.; Dybek, A.; Pietraszewska, D.; Skucha-Nowak, M.; Postek-Stefanska, L. Management of teeth affected by molar incisor hypomineralization using a resin infiltration technique- A systematic review. Coatings 2022, 12, 964. [CrossRef]
93. Kim, S.; Kim, E.Y.; Jeong, T.S.; Kim, J.W. The evaluation of resin infiltration for masking labial enamel white spot lesions. Int. J. Paediatr. Dent. 2011, 21, 241–248. [CrossRef]
94. Somani, C.; Taylor, G.D.; Garot, E.; Rouas, P.; Lygidakis, N.A.;Wong, F.S.L. An update of treatment modalities in children and adolescents with teeth affected by molar incisor hypomineralisation (MIH): A systematic review. Eur. Arch. Paediatr. Dent. 2022, 23, 39–64. [CrossRef] [PubMed]
95. Elbaz, G.A.; Mahfouz, S.M. Efficacy of two different treatment modalities on masking white spot lesions in children with molar incisor hypomineralization. Egypt. Dent. J. 2017, 63, 2147–2154. [CrossRef]
96. Bhandari, R.; Thakur, S.; Singhal, P.; Chauhan, D.; Jayam, C.; Jain, T. Concealment effect of resin infiltration on incisor of grade I molar incisor hypomineralization patients: An in vivo study. J. Conserv. Dent. 2018, 21, 450–454. [CrossRef] [PubMed]
97. Kielbassa, A.M.; Leimer, M.R.; Hartmann, J.; Harm, S.; Pasztorek, M.; Ulrich, I.B. Ex vivo investigation on internal tunnel approach/internal resin infiltration and external nanosilver-modified resin infiltration of proximal caries exceeding into dentin. PLoS ONE 2020, 15, e0228249. [CrossRef]
98. Schneider, H.; Park, K.J.; Rueger, C.; Ziebolz, D.; Krause, F.; Haak, R. Imaging resin infiltration into non-cavitated carious lesions by optical coherence tomography. J. Dent. 2017, 60, 94–98. [CrossRef]
99. Yim, H.K.; Min, J.H.; Kwon, H.K.; Kim, B.I. Modification of surface pretreatment of white spot lesions to improve the safety and efficacy of resin infiltration. Korean J. Orthod. 2014, 44, 195–202. [CrossRef]
100. Mandava, J.; Reddy, Y.S.; Kantheti, S.; Chalasani, U.; Ravi, R.C.; Borugadda, R.; Konagala, R.K. Microhardness and penetration of artificial white spot lesions treated with resin or colloidal silica infiltration. J. Clin. Diagn. Res. 2017, 11, ZC142–ZC146. [CrossRef]
101. Theodory, T.G.; Kolker, J.L.; Vargas, M.A.; Maia, R.R.; Dawson, D.V. Masking and penetration ability of various sealants and icon in artificial initial caries lesions in vitro. J. Adhes. Dent. 2019, 21, 265–272.
102. Paris, S.; Bitter, K.; Naumann, M.; Dörfer, C.E.; Meyer-Lueckel, H. Resin infiltration of proximal caries lesions differing in ICDAS codes. Eur. J. Oral Sci. 2011, 119, 182–186. [CrossRef]
103. Liang, Y.; Deng, Z.; Dai, X.; Tian, J.; Zhao,W. Micro-invasive interventions for managing non-cavitated proximal caries of different depths: A systematic review and meta-analysis. Clin. Oral Invest. 2018, 22, 2675–2684. [CrossRef]
104. Soviero, V.M.; Paris, S.; Leal, S.C.; Azevedo, R.B.; Meyer-Lueckel, H. Ex vivo evaluation of caries infiltration after different application times in primary molars. Caries Res. 2013, 47, 110–116. [CrossRef] [PubMed]
105. Aswani, R.; Chandrappa, V.; Uloopi, K.S.; Chandrasekhar, R.; Roja Ramya, K.S. Resin infiltration of artificial enamel lesions: Evaluation of penetration depth, surface roughness and color stability. Int. J. Clin. Pediatr. Dent. 2019, 12, 520–523. [PubMed]
106. Schwendicke, F.; Tzschoppe, M.; Paris, S. Radiographic caries detection: A systematic review and meta-analysis. J. Dent. 2015, 43, 924–933. [CrossRef] [PubMed]
107. Watts, D.; McCabe, J. Aluminium radiopacity standards for dentistry: An international survey. J. Dent. 1999, 27, 73–78. [CrossRef] [PubMed]
108. Moeinian, M. Development of a Radiopaque Infiltration Resin for Early Enamel Carious Lesion. PhD Thesis, Queen Mary University of London, London, UK, April 2018.
109. Polak-Kowalska, K.; Pels, E. In vitro and in vivo assessment of enamel colour stability in teeth treated with low-viscosity resin infiltration–a literature review. J. Stoma. 2019, 72, 135–141. [CrossRef]
110. Zhao, X.; Ren, Y.F. Surface properties and color stability of resin-infiltrated enamel lesions. Oper. Dent. 2016, 41, 617–626. [CrossRef]
111. Alqahtani, S.; Abusaq, A.; Alghamdi, M.; Shokair, N.; Albounni, R. Colour stability of resin infiltrated white spot lesion after exposure to stain-causing drinks. Saudi J. Biol. Sci. 2022, 29, 1079–1084. [CrossRef]
112. Borges, A.; Caneppele, T.; Luz, M.; Pucci, C.; Torres, C. Color stability of resin used for caries infiltration after exposure to different staining solutions. Oper. Dent 2014, 39, 433–440. [CrossRef]
113. Ceci, M.; Rattalino, D.; Viola, M.; Beltrami, R.; Chiesa, M.; Colombo, M.; Poggio, C. Resin infiltrant for non-cavitated caries lesions: Evaluation of color stability. J. Clin. Exp. Dent. 2017, 9, e231–e237. [CrossRef]
114. Furuse, A.Y.; Fernandes Neto, C.; Guimarães, G.M.D.F.; Terrabuio, B.R.; Rizzante, F.A.P.; Wang, L. Color evaluation of white spot lesions treated with resin infiltration after water or grape juice storage. Braz. J. Oral. Sci. 2020, 19, e201674. [CrossRef]
115. Cohen-Carneiro, F.; Pascareli, A.M.; Christino, M.R.; Vale, H.F.; Pontes, D.G. Color stability of carious incipient lesions located in enamel and treated with resin infiltration or remineralization. Int. J. Paediatr. Dent. 2014, 24, 277–285. [CrossRef] [PubMed]
116. Araújo, G.S.A.; Naufel, F.S.; Alonso, R.C.B.; Lima, D.A.N.L.; Puppin-Rontani, R.M. Influence of staining solution and bleaching on color stability of resin used for caries infiltration. Oper. Dent. 2015, 40, e250–e256. [CrossRef] [PubMed]
117. Teughels,W.; Van Assche, N.; Sliepen, I.; Quirynen, M. Effect of material characteristics and/or surface topography on biofilm development. Clin. Oral Implants Res. 2006, 17, 68–81. [CrossRef] [PubMed]
118. Gurdogan, E.B.; Ozdemir-Ozenen, D.; Sandalli, N. Evaluation of surface roughness characteristics using atomic force microscopy and inspection of microhardness following resin infilration. J. Esthet. Restor. Dent. 2017, 29, 201–208. [CrossRef]
119. Ulrich, I.; Mueller, J.; Wolgin, M.; Frank, W.; Kielbassa, A.M. Tridimensional surface roughness analysis after resin infiltration of (deproteinized) natural subsurface carious lesions. Clin. Oral Investig. 2015, 19, 1473–1483. [CrossRef]
120. Leland, A.; Akyalcin, S.; English, J.D.; Tufekci, E.; Paravina, R. Evaluation of staining and color changes of resin infiltration system. Angle Orthod. 2016, 86, 900–904. [CrossRef]
121. Jansen, E.E.; Meyer-Lueckel, H.; Esteves-Oliveira, M.; Wierichs, R.J. Do bleaching gels affect the stability of the masking and caries-arresting effects of caries infiltration—In vitro. Clin. Oral Invest. 2021, 25, 4011–4021. [CrossRef]
122. Meyer-Lueckel, H.; Wardius, A.; Krois, J.; Bitter, K.; Moser, C.; Paris, S.; Wierichs, R.J. Proximal caries infiltration-Pragmatic RCT with 4 years of follow-up. J. Dent. 2021, 111, 103733. [CrossRef]
123. Tiskaya, M.; Shahid, S.; Gillam, D.; Hill, R. The use of bioactive glass (BAG) in dental composites: A critical review. Dent. Mater. 2021, 37, 296–310. [CrossRef]
124. Van Landuyt, K.L.; Snauwaert, J.; De Munck, J.; Peumans, M.; Yoshida, Y.; Poitevin, A.; Coutinho, E.; Suzuki, K.; Lambrechts, P.; van Meerbeek, B. Systematic review of the chemical composition of contemporary dental adhesives. Biomaterials 2007, 28, 3757–3785. [CrossRef]
125. Hashemian, A.; Shahabi, S.; Behroozibakhsh, M.; Najafi, F.; Abdulrazzaq Jerri Al-Bakhakh, B.; Hajizamani, H. A modified TEGDMA-based resin infiltrant using polyurethane acrylate oligomer and remineralising nano-fillers with improved physical properties and remineralisation potential. J. Dent. 2021, 113, 103810. [CrossRef] [PubMed]
126. Sideridou, I.; Tserki, V.; Papanastasiou, G. Study of water sorption, solubility and modulus of elasticity of light-cured dimethacrylate-based dental resins. Biomaterials 2003, 24, 655–665. [CrossRef] [PubMed]
127. Prodan, D.; Moldovan, M.; Chisnoiu, A.M.; Saros, i, C.; Cuc, S.; Filip, M.; Gheorghe, G.F.; Chisnoiu, R.M.; Furtos, G.; Cojocaru, I.; et al. Development of new experimental dental enamel resin infiltrants- Synthesis and characterization. Materials 2022, 15, 803. [CrossRef] [PubMed]
128. Sfalcin, R.A.; Correr, A.B.; Morbidelli, L.R.; Araújo, T.G.F.; Feitosa, V.P.; Correr-Sobrinho, L.; Watson, T.F.; Sauro, S. Influence of bioactive particles on the chemical-mechanical properties of experimental enamel resin infiltrants. Clin. Oral Investig. 2017, 21, 2143–2151. [CrossRef]
129. Dai, Z.; Xie, X.; Zhang, N.; Li, S.; Yang, K.; Zhu, M.; Weir, M.D.; Xu, H.H.K.; Zhang, K.; Zhao, Z.; et al. Novel nanostructured resin infiltrant containing calcium phosphate nanoparticles to prevent enamel white spot lesions. J. Mech. Behav. Biomed. Mater. 2022, 126, 104990. [CrossRef]
130. Obeid, A.T.; Garcia, L.H.A.; Nascimento, T.R.L.; Castellano, L.R.C.; Bombonatti, J.F.S.; Honório, H.M.; Mondelli, R.F.L.; Sauro, S.; Velo, M.M.A.C. Effects of hybrid inorganic-organic nanofibers on the properties of enamel resin infiltrants-An in vitro study. J. Mech. Behav. Biomed. Mater. 2022, 126, 105067. [CrossRef]
131. Skucha-Nowak, M.; Machorowska-Pienia˛z˙ ek, A.; Tanasiewicz, M. Assessing the penetrating abilities of experimental preparation with dental infiltrant features using optical microscope: Preliminary study. Adv. Clin. Exp. Med. 2016, 25, 961–969. [CrossRef]
132. Inagaki, L.T.; Alonso, R.C.; Araújo, G.A.; de Souza-Junior, E.J.; Anibal, P.C.; Höfling, J.F.; Pascon, F.M.; Puppin-Rontani, R.M. Effect of monomer blend and chlorhexidine-adding on physical, mechanical and biological properties of experimental infiltrants. Dent. Mater. 2016, 32, e307–e313. [CrossRef]
133. Flor-Ribeiro, M.D.; Graziano, T.S.; Aguiar, F.H.B.; Stipp, R.N.; Marchi, G.M. Effect of iodonium salt and chitosan on the physical and antibacterial properties of experimental infiltrants. Braz. Oral Res. 2019, 33, e075. [CrossRef]
134. Yu, J.; Huang, X.; Zhou, X.; Han, Q.; Zhou, W.; Liang, J.; Xu, H.H.K.; Ren, B.; Peng, X.; Weir, M.D.; et al. Anti-caries effect of resin infiltrant modified by quaternary ammonium monomers. J. Dent. 2020, 97, 103355. [CrossRef]
135. Cuppini, M.; Garcia, I.M.; de Souza, V.S.; Zatta, K.C.; Visioli, F.; Leitune, V.C.B.; Guterres, S.S.; Scholten, J.D.; Collares, F.M. Ionic liquid-loaded microcapsules doped into dental resin infiltrants. Bioact. Mater. 2021, 6, 2667–2675. [CrossRef] [PubMed]
136. Fischer, M.; Skucha-Nowak, M.; Chmiela, B.; Korytkowska-Wałach, A. Assessment of the potential ability to penetrate into the hard tissues of the root of an experimental preparation with the characteristics of a dental infiltrant, enriched with an antimicrobial component- Preliminary study. Materials 2021, 14, 5654. [CrossRef] [PubMed]
137. Gindri, I.M.; Siddiqui, D.A.; Frizzo, C.P.; Martins, M.A.P.P.; Rodrigues, D.C. Improvement of tribological and anti-corrosive performance of titanium surfaces coated with dicationic imidazolium-based ionic liquids. RSC Adv. 2016, 6, 78795–78802. [CrossRef]
138. Garcia, I.M.; Ferreira, C.J.; de Souza, V.S.; Leitune, V.C.B.; Samuel, S.M.W.; Balbinot, G.D.S.; de Motta, A.D.S.; Visioli, F.; Scholten, J.D.; Collares, F.M. Ionic liquid as antibacterial agent for an experimental orthodontic adhesive. Dent. Mater. 2019, 35, 1155–1165. [CrossRef]
139. Liu, Y.; Zhao, Q. Influence of surface energy of modified surfaces on bacterial adhesion. Biophys. Chem. 2005, 117, 39–45. [CrossRef]
140. Collares, F.M.; Garcia, I.M.; Bohns, F.R.; Motta, A.; Melo, M.A.; Leitune, V.C.B. Guanidine hydrochloride polymer additive to undertake ultraconservative resin infiltrant against Streptococcus mutans. Eur. Polym. J. 2020, 133, 109746. [CrossRef]
141. Ebrahimi, M.; Janani, A.; Majidinia, S.; Sadeghi, R.; Shirazi, A.S. Are self-etch adhesives reliable for primary tooth dentin? A systematic review and meta-analysis. J. Conserv. Dent. 2018, 21, 243–250.
142. Wang, L.; Freitas, M.C.C.A.; Prakki, A.; Mosquim, V.; González, A.H.M.; Rios, D.; Honorio, H.M. Experimental self-etching resin infiltrants on the treatment of simulated carious white spot lesions. J. Mech. Behav. Biomed. Mater. 2021, 113, 104146. [CrossRef]
143. Pedreira, P.R.; Damasceno, J.E.; Mathias, C.; Sinhoreti, M.; Aguiar, F.; Marchi, G.M. Influence of incorporating zirconium- and barium-based radiopaque filler into experimental and commercial infiltrants. Oper. Dent. 2021, 46, 566–576. [CrossRef]
144. Nowak-Wachol, A.; Korytkowska-Wałach, A.; Chmiela, B.; Wachol, K.; Łopaci´ nski, M.; Wyszy´ nska, M.; Al-Dulaimi, Y.; Skucha-Nowak, M. Yttrium trifluoride as a marker of infiltration rate of decalcified root cementum: An in vitro study. Polymers 2022, 14, 780. [CrossRef]
145. Askar, H.; Lausch, J.; Dörfer, C.E.; Meyer-Lueckel, H.; Paris, S. Penetration of micro-filled infiltrant resins into artificial caries lesions. J. Dent. 2015, 43, 832–838. [CrossRef] [PubMed]
146. Askar, H.; Schwendicke, F.; Lausch, J.; Meyer-Lueckel, H.; Paris, S. Modified resin infiltration of non-, micro- and cavitated proximal caries lesions in vitro. J. Dent. 2018, 74, 56–60. [CrossRef] [PubMed]