On the pBuM189 satellite DNA variability among South American populations of Drosophila buzzatii
The pBuM189 satellite DNA was analysed in Drosophila buzzatii populations that cover most of the species distribution in South America. This satDNA consists of A+T-rich monomers of 189 bp and previous studies showed a fast rate of evolutionary change of this component of D. buzzatii genome. A total of 63 pBuM189 repetition units from 14 D. buzzatii populations (9 from Brazil and 5 from Argentina) were studied. The average nucleotide variability among the 63 repeats is 4.2 %. At least one repeat (Juan/4) seems to be part of another pBuM189 satDNA subfamily. The nucleotide alignment of all 63 repeats revealed no specific nucleotide substitutions, or indels, that could discriminate each population or groups of geographically close populations. Such lack of satDNA interpopulational differentiation is congruent with previous mtDNA data that indicate a high gene flow and very little population differentiation throughout most of the D. buzzatii distribution in South America. Gene flow might have been possible during glaciation events in the Pleistocene, such as the one occurred between 13 000 and 18 000 years ago, when D. buzzatii probably had a more continuous distribution than what is observed today.
The cactophilic fly Drosophila buzzatii (buzzatii cluster, repleta group) has a wide distribution in South America, being found throughout most territories of Argentina, Bolivia, Paraguay and Brazil. The species uses decaying tissues of several prickly pear cacti (Opuntia ssp.) and columnar cacti for feeding and breeding (PEREIRA et al. 1983). During the last two hundred years, Opuntia cacti have been transported by man to several regions of the world, including countries of the Mediterranean basin, Africa and Australia. Consequently, D. buzzatii migrated with its hosts. This situation made D. buzzatii an appropriate organism for addressing questions related to genetic changes during the colonization process (FONTDEVILA et al. 1982; HALLIBURTON and BARKER 1993; ROSSI et al. 1996; FRYDENBERG et al. 2002).
According to chromosomal inversion data, the Argentinean Chaco is the most probable centre of its radiation (WASSERMAN 1962; CARSON and WASSERMAN 1965). D. buzzatii is also very abundant in the Chaco, associated with a number of different species of cacti (Vilela et al. 1980). In general, Brazilian populations are patchily distributed throughout small areas of xerophytic vegetation, where they have been found at very low frequencies (VILELA et al. 1983; TIDON-SKLORZ et al. 1994; TIDON-SKLORZ and SENE 1995). The only exception is southern Brazil, where relatively large populations were found (VILELA et al. 1983; RUIZ et al. 2000).
It has been postulated that D. buzzatii colonized Brazil through changes in the arid vegetation distribution during glaciation events, such as those occurred in late Pleistocene (BARKER et al. 1985; FIGUEIREDO and SENE 1992).
BAIMAI et al. (1983) analysed the metaphase chromosomes of several D. buzzatii populations from Argentina and Brazil. All sampled populations exhibit the same metaphase plate. BARKER et al. (1985), found no significant allozyme differences among South American populations. Only a small local differentiation seemed to have happened in the Brazilian populations from Bahia (northeast) and Tramanda´1 (south). In another study, FIGUEIREDO and SENE (1992) reported that from 16 chromosome inversions detected in Argentina, only two were present in the Brazilian populations and no one was found in northeastern Brazil. ROSSI et al. (1996) and DE BRITO et al. (2002) found high levels of mtDNA uniformity among South American D. buzzatii populations and several tests indicated high gene flow throughout most of D. buzzatii geographical distribution. DE BRITO et al. (2002) also calculated that D. buzzatii has been present in Brazil for at least 100 thousands years, suggesting a pre-Holocene expansion of D. buzzatii Argentinean populations towards Brazil.
In order to get more information on the genetic variability of D. buzzatii in South America, we looked for a nuclear DNA. Satellite DNA (satDNA) is a class of highly repetitive non-coding DNA that is tandemly arranged in large homogeneous arrays in the genome of nearly all eukaryotic organisms. The usually fast rate of evolutionary change of satDNA sequences is supported by a number of satDNA families that proved to be species-specific (BACH- MANN et al. 1989; KING and CUMMINGS 1997).
Previous studies showed that satDNA sequences might be useful in the discrimination of conspecific populations (ELDER and TURNER 1994; WU et al. 1999).
KUHN et al. (1999) described the pBuM189 satellite DNA of D. buzzatii. It consists of repetition units slightly AT-rich and 189 bp long. The five described pBuM189 sequences were obtained from the genomic DNA of only one D. buzzatii population. Hybridisation and PCR experiments failed to detect pBuM189 sequences in the genome of closely related species such as D. serido, D. borborema or D. koepferae. Therefore, the available data point to a fast rate of evolutionary change of this component of D. buzzatii nuclear DNA.
In the present study, we analysed 63 pBuM189 sequences from strains representing 14 D. buzzatii populations (9 Brazilian and 5 Argentinean). The populations cover most of the geographical distribution of this species in South America.
Material and methods
Samples
The geographical location of all D. buzzatii populations sampled is shown in Fig. 1. The strains used with their respective localities are: D69R5 (1); J79H41 (2); D54F5 (3); H86G8 (4); D42F2 (5); H99X6 (6); H42F1 (7); J25A20 (8); J28E15 (9); ArgE3 (10); Cat (11), Hondo (12); Salta (13) and Ticucho (14).
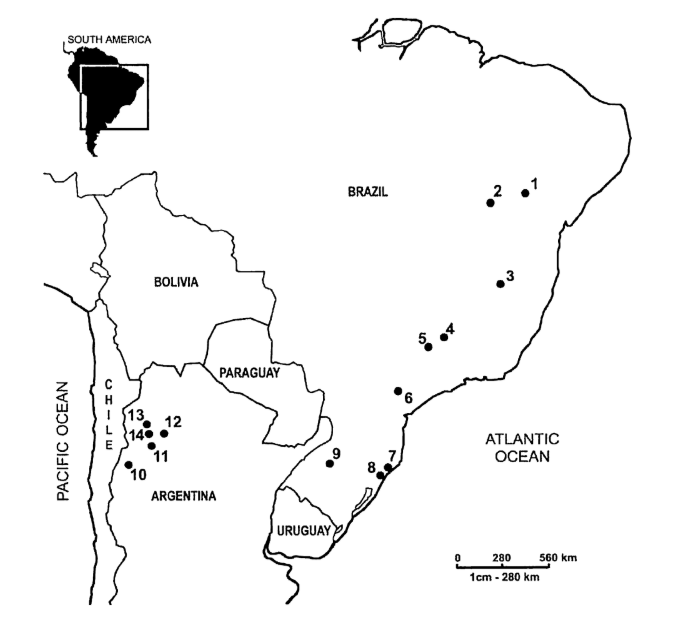
Molecular techniques
The pBuM189 sequences were originally isolated from the genome of D. buzzatii after DNA digestion with the MspI enzyme (KUHN et al. 1999). In the present work, the SspI enzyme (which is also present only once per pBuM189 repetition unit) was used in order to facilitate the cloning procedure. pBuM189 satDNA fragments were identified by their appearance as prominent bands of approximately 190 bp in the background smear of genomic DNA. The DNA from the 190 bp DNA band was eluted from the gel by overnight incubation in 500mM NaAc; 1mM EDTA. The recovered fragments were ligated into a pUC18 plasmid vector and transformed to competent Escherichia coli DH5-a cells. Recombinant clones were selected as white colonies on ampicillin plates containing X-gal (5-bromo-4-chloro-3-indolyl-β-D- galactoside) and IPTG (isopropyl-β-D-thiogalactopyranoside). The template DNA reaction for sequencing was prepared according to the BigDye Terminator Cycle Sequencing Ready Reaction Kit (Perkin-Elmer) manual. Automatic DNA sequencing was performed on an ABI Prism™ 377 (Perkin-Elmer) sequencer.
The MEGA 2.1 program (KUMAR et al. 2001) was used for the estimation of nucleotide variability, genetic distances and construction of a NJ dendrogram.
Results and discussion
As it was already expected from previously pBuM189 sequence data, genomic DNA digestion of 13 D. buzzatii strains with SspI produced a heavily stained DNA band of approximately 190 bp, corresponding to pBuM189 monomers, followed by bands of decreasing intensity signals of 380 bp (dimers), 570 bp (trimers), and so on fragments. The 190 bp DNA band from each digestion was independently eluted from the gel and cloned. A total of 58 clones were sequenced, 39 from 8 Brazilian populations (localities 1 – 4, 6 – 9) and 19 from 5 Argentinean populations (localities 10 – 14). The nucleotide alignment of these 58 clones with the 5 pBuM189 repeats (sert/1 – 5 in the present work) from locality (5) described earlier by KUHN et al. (1999) proved that they all represent pBuM189 satDNA monomers (Fig. 2).
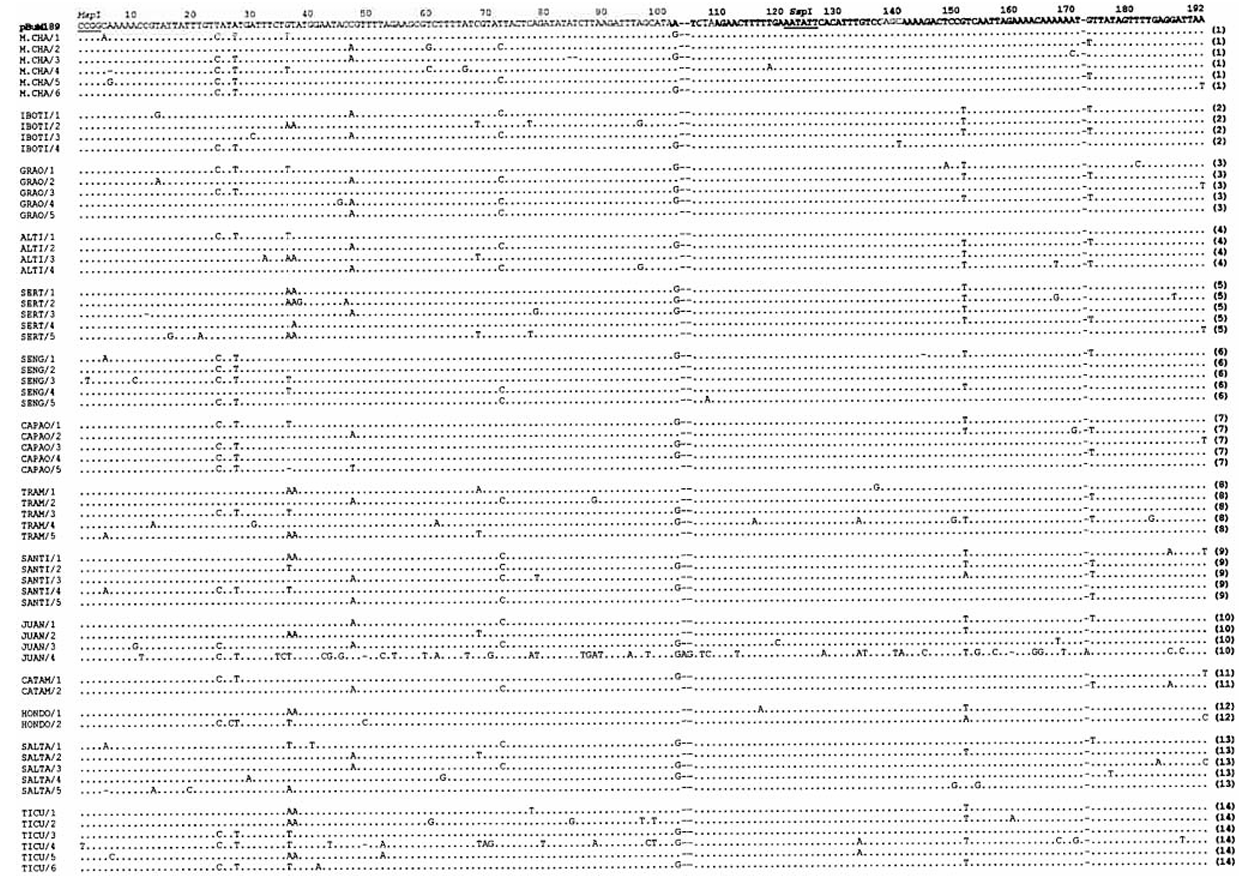
The 63 pBuM189 repeats are slightly A+T-rich (71 %) and the average sequence variability is 4.2 %. The main source of variation among the 63 pBuM189 repeats are single nucleotide substitutions, being 69 % repeat-specific and the remaining common to two or more repeats. Only 11 indels were found.
One pBuM189 repeat seems to be exceptional. The Juan/4 clone from locality (10) exhibited unexpected high levels of sequence divergence in all pairwise comparisons (22.1 % on average and always exceeding 19 %). Moreover, while typical pBuM189 sequences present on average 1.7 % repeat-specific nucleotide substitutions, 30 were found in the Juan/4 repeat (e.g. T at positions 11 and 42). Such high degree of nucleotide divergence suggests that the Juan/4 repeat might belong to another pBuM189 subfamily. From a theoretical viewpoint, the origin of satDNA subfamilies depends on variables such as copy number, genomic distribution of the satDNA family and the relative rates of non-reciprocal exchanges within and between non-homologous chromosomes (DOVER 1986).
If the Juan/4 repeat is excluded from the sample, the average nucleotide variability of the remaining 62 pBuM189 sequences decreases to 3.7 %. This value is almost identical to the 3.8 % calculated by KUHN et al. (1999) based on five pBuM189 sequences isolated from only one D. buzzatii population (locality 5).
Among the other pBuM189 repeats, Ticu/4 from locality (14) is the most similar to Juan/4 for presenting two specific nucleotides (G at position 70 and A at position 88) and one indel (position 49) that are otherwise exclusive to the Juan/4 repeat and several other substitutions in common to Juan/4 and other repeats (e.g. T at position 36 or A at position 133). The Ticu/4 repeat itself is the most divergent pBuM189 repeat (10.2 % on average) after Juan/4, presenting 6 repeat-specific nucleotide substitutions (positions 1, 69, 79, 97, 98, 167 and 188). Therefore, the Ticu/4 must be considered as an intermediate evolutionary step between ‘‘typical'' pBuM189 repeats and the highly diverged Juan/4 repeat. Interestingly, both Juan/4 and Ticu/4 rare repeats are from Argentina, where the species probably originated (WASSERMAN 1962; CARSON and WASSERMAN 1965).
According to theoretical and empirical data, differentiation of satDNA sequences should arise according to the concept of concerted evolution (DOVER 1986; ELDER and TURNER 1994). In other words, satDNA arrays from different populations or species might be homogenised for different mutations by the effect of molecular mechanisms such as unequal crossing-over and gene conversion coupled to genetic isolation and genetic drift.
The nucleotide alignment of the 63 pBuM189 repeats from 14 D. buzzatii populations (4.5 % repeats on average per population) revealed no specific nucleotide substitution, or indel, that could discriminate a specific population or groups of geographically close populations (Fig. 2). In some cases, more than two clones shared identical nucleotide sequence, as in the case of the Capao/3, Grao/3, M.Cha/6 and Catam/1 or M.Cha/1 and Santi/4 clones, but they are derived from quite distant geographic localities. On the other hand, no identical repeats were found in a single population.
Genetic distances among the 63 pBuM189 nucleotide sequences were calculated according to the Kimura's two parameter method (KIMURA 1980) and a ‘‘neighbor joining'' dendrogram (SAITOU and NEI 1987) was constructed (Fig. 3). The Juan/4 sequence was located in a clearly separate branch from the other pBuM189 repeats, supporting the assumption that it belongs to another pBuM189 satDNA subfamily. The Ticu/4 repeat was located in a branch between the Juan/4 and the remaining 61 pBuM189 repeats. Within the remaining 61 pBuM189 repeats, no cluster containing pBuM189 sequences from one population or groups of geographically close populations could be observed.
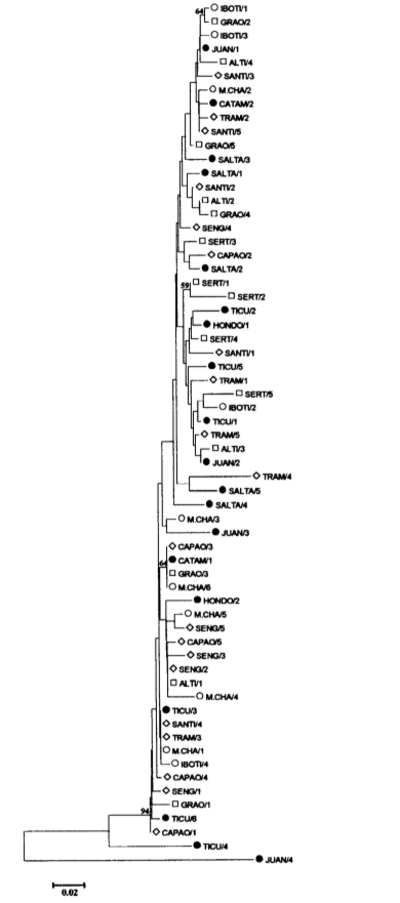
Altogether, the data indicate high levels of pBuM189 sequence similarity among D. buzzatii populations that are located, in some cases, more than 3000 km apart. Such lack of satDNA interpopulational differentiation is congruent to mtDNA data that indicate a high gene flow and very little population substructure throughout most of the D. buzzatii distribution in South America (ROSSI et al. 1996; DE BRITO et al. 2002). Accordingly, there must be an adaptative explanation for the loss of chromosomal inversion polymorphism in Brazilian populations (compared to Argentinean populations), reported by FIGUEIREDO and SENE (1992).
Currently, the Chaco (northern Argentina and east-Bolivia) and the Caatinga (northeast Brazil) are the only two extensive areas of arid vegetation in South America, presenting a high density and diversity of cactus species. In other areas, cactacea are less frequent and patchily distributed. The same is true for D. buzzatii populations, which depend on cactus for feeding and breeding. It is widely accepted that global climate underwent recurrent cycles of cold-dry and hot-wet weather during the Pleistocene, when at least four major glacial events, and many minor ones, occurred. For instance, in the last glaciation event (18 000 to 13 000 years ago), the climate in South America was dry and the Chaco was connected to the Caatingas through xerophytic formations in the central Brazil and Atlantic coast (AB'SABER 1977). Consequently, D. buzzatii probably had a much more continuous distribution than what is observed today. Such situation might have allowed the gene flow among South American D. buzzatii populations in a large degree.
To date, most satellite DNAs described in the literature were isolated from individuals from a single strain or locality. The present data is one of the few examples that provide information on the satDNA sequence variability among natural populations of a widespread species.
Authors: Gustavo C. S. Kuhn, Fernando F. Franco, Wilson A. Silva Jr, Nilce M. Martinez-Rossi and Fabio M. Sene
References:
- Ab'Saber, N. A. 1977. Espac¸os ocupados pela expansão dos climas secos da América do Sul, por ocasião dos períodos glaciais quaternários. – Paleoclimas 3: 1 – 19.
- Bachmann, L., Raab, M. and Sperlich, D. 1989. Satellite DNA and speciation: a species specific satellite DNA of Drosophila guanche. – Z. Zool. Syst. Evol. Forsch. 27: 84 – 93.
- Baimai, V., Sene, F. M. and Pereira, M. A. Q. R. 1983. Heterochromatin and karyotypic differentiation of some neotropical cactus-breeding species of the Drosophila repleta group. – Genetica 67: 81 – 92.
- Barker, J. S. F., Sene, F. M., East, P. P. et al. 1985. Allozyme and chromossomal polymorphism of Drosophila buzzatii in Brazil and Argentina. – Genetica 67: 161 – 170.
- Carson, H. L. and Wasserman, M. 1965. A widespread chromosomal polymorphism in a widespread species, Drosophila buzzatii. – Am. Nat. 99: 111 – 115.
- De Brito, R. A., Manfrin, M. H. and Sene, F. M. 2002. Mitochondrial DNA phylogeography of Brazilian populations of Drosophila buzzatii. – Genet. Mol. Biol. 2: 161 – 171.
- Dover, G. A. 1986. Molecular drive in multigene families: how biological novelties arise, spread and are assimilated. – Trends Genet. 2: 159 – 165.
- Elder, F. J. and Turner, B. J. 1994. Concerted evolution at the population level: pupfish HindIII satellite DNA sequences. – Proc. Natl Acad. Sci. 91: 994 – 998.
- Figueiredo, V. L. C. and Sene, F. M. 1992. Chromosome variability in Brazilian populations of Drosophila buzzatii (Diptera, Drosophilidae). – Revta. Bras. Biol. 52: 555 – 561.
- Fontdevila, A., Ruiz, A., Ocana, J. et al. 1982. The evolutionary history of Drosophila buzzatii. II. How much has chromosomal polymorphism changed in colonization? – Evolution 36: 843 – 851.
- Frydenberg, J., Pertoldi, C., Dahlgaard, J. et al. 2002. Genetic variation in original and colonizing Drosophila buzzatii populations analysed by microsatellite loci isolated with a new PCR screening method. – Mol. Ecol. 11: 181 – 190.
- Halliburton, R. and Barker, J. S. F. 1993. Lack of mitochondrial DNA variation in Australian Drosophila buzzatii. – Mol. Biol. Evol. 10: 484 – 487.
- Kimura, M. 1980. A single method for estimating evolutionary rate of base substitutions through comparative studies of nucleotide sequences. – J. Mol. Evol. 16: 111 – 120.
- King, L. M. and Cummings, M. P. 1997. Satellite DNA repeat sequence variation is low in three species of the burying beetles in the genus Nicrophorus (Coleoptera: Silphidae). – Mol. Biol. Evol. 14: 1088 – 1095.
- Kuhn, G. C. S., Bollgönn, S., Sperlich, D. et al. 1999. Characterization of a species-specific satellite DNA of Drosophila buzzatii. – J. Zool. Syst. Evol. Res. 37: 109 – 112.
- Kumar, S., Tamura, K., Jakobsen, I. B. et al. 2001. MEGA2: molecular evolutionary genetics analysis software. – Arizona State Univ.
- Pereira, M. A. Q. R., Vilela, C. R. and Sene, F. M. 1983. Notes on breeding and feeding sites of some species of the repleta group of the genus Drosophila (Diptera, Drosophilidae). – Ciência e Cultura 35: 1313 – 1319.
- Rossi, M.S., Barrio, E., Latorre, A. et al. 1996. The evolutionary history of Drosophila buzzatii. XXX. Mitochondrial DNA polymorphism in original and colonizing populations. – Mol. Biol. Evol. 13: 314 – 323.
- Ruiz, A., Cansian, A. M., Kuhn, G. C. S. et al. 2000. The Drosophila serido speciation puzzle: putting new pieces together. – Genetica 108: 217 – 227.
- Saitou, N. and Nei, M. 1987. The neighbor-joining method: a new method for reconstructing phylogenetic trees. – Mol. Biol. Evol. 4: 406 – 425.
- Tidon-Sklorz, R. and Sene, F. M. 1995. Fauna of Drosophila (Diptera, Drosophilidae) in the northern area of the ‘‘Cadeia do Espinhac¸o'', states of Minas Gerais and Bahia, Brazil: biogeographical and ecological aspects. – Iheringia Ser. Zool. 78: 85 – 94.
- Tidon-Sklorz, R., Vilela, C. R., Sene, F. M. et al. 1994. The genus Drosophila (Diptera, Drosophilidae) in the Serra do Cipó, state of Minas Gerais, Brazil. – Revta. Bras. Ent. 38: 627 – 637.
- Vilela, C. R., Sene, F. M. and Pereira, M. A. Q. R. 1980. On the Drosophila fauna of Chaco and east slopes of the Andes in Argentina. – Revta. Bras. Biol. 40: 837 – 841. Vilela, C. R., Pereira, M. A. Q. R. and Sene, F. M. 1983. Preliminary data on the geographical distribution of Drosophila species within morphoclimatic domains of Brazil. II. The repleta group. – Ciên. Cult. São Paulo 35: 66 – 70.
- Wasserman, M. 1962. Cytological studies of the repleta group of the genus Drosophila. V. The mulleri subgroup. – Univ. Texas Publ. 6205: 85 – 118.
- Wu, W. L., Wang, J. P., Tseng, M. C. et al. 1999. Cloning and genetic variability of a HindIII repetitive DNA in Acrossocheilus paradoxus (Cyprinidae). – Genome 42: 780 – 788.