Evaluation of the Shaping Characteristics of ProTaper Gold, ProTaper NEXT, and ProTaper Universal in Curved Canals
Abstract
Introduction: This study evaluated the shaping characteristics of the ProTaper Gold system (PTG; Dentsply Maillefer, Ballaigues, Switzerland) and compared it with that of the ProTaper Next (PTN, Dentsply Maillefer) and ProTaper Universal (PTU, Dentsply Maillefer) systems using micro–computed tomographic imaging.
Methods: Twenty-four mandibular first molars with 2 separate mesial canals were matched anatomically using micro–computed tomographic scanning (Sky- Scan1174v2; Bruker-microCT, Kontich, Belgium) with a voxel size of 19.6 mm. Canals were prepared with PTG, PTU, or PTN rotary systems to F2 or X2 instruments, respectively, and scanned again. Coregistered images were evaluated for 2- and 3-dimensional morphometric measurements of canal transportation, centering ability, untouched canal walls, and remaining dentin thickness. Data were statistically compared using Kruskal-Wallis and 1-way analysis of variance tests (a = 5%).
Results: Overall, PTN showed significantly higher percentage values of static voxels than PTG and PTU systems (P < .05). Surface area, perimeter, and minor diameter were higher in the PTG and PTU groups than in the PTN group (P < .05). No difference was observed in form factor, roundness, major diameter, aspect ratio, or structure model index (P > .05). PTG (0.11 ± 0.05 mm) and PTN (0.09 0.05 mm) produced significantly less transportation than PTU (0.14 ± 0.07 mm) (P < .05), and the percentage decrease in dentin thickness was significantly lower for PTG (22.67 ± 2.96) and PTN (17.71 ± 5.93%) (P ≥ .05) than PTU (29.93 ± 6.24%) (P < .05).
Conclusions: PTG and PTN produced less transportation and maintained more dentin than PTU. PTN had less canal wall contact than PTG and PTU, but all file systems were able to instrument moderately curved mesial root canals of mandibular molars without clinically significant errors. (J Endod 2015;■:1–7)
Apical periodontitis is caused by root canal infection. Its treatment is focused on the elimination of microorganisms by chemomechanical preparation of the root canal. Nickel-titanium (NiTi) rotary instruments used for this purpose produce a more centered preparation of the canal, with less transportation than stainless steel instruments. NiTi rotary instrument designs continue evolving to optimize their cutting and shaping characteristics. With many new systems available on the market, clinicians require an impartial evaluation of these systems’ characteristics to help the select systems to use clinically.
ProTaper Next (PTN; Dentsply Maillefer, Ballaigues, Switzerland) is a relatively new system. PTN instruments are made of M-wire, a unique NiTi alloy manufactured by a thermal treatment process that reportedly increases flexibility and resistance to cyclic fatigue. These instruments incorporate a variable regressive taper design, unique offset mass of rotation, and rectangular cross section, which according to the manufacturer are designed to reduce points of contact with the canal walls generating less fatigue in the instrument during use.
ProTaper Universal (PTU, Dentsply Maillefer) and ProTaper Gold (PTG, Dentsply Maillefer) systems share an identical instrument design with a triangular cross section and a variable progressive taper. PTG is manufactured by proprietary metallurgy that reportedly increases its flexibility and its resistance to cyclic fatigue. To our knowledge, research data on the shaping characteristics of PTG were not yet available at the time this study was undertaken. Thus, this study aimed to evaluate the shaping characteristics of the PTG system and compare it with that of the PTN and PTU systems using micro–computed tomographic (micro-CT) imaging.
Materials and Methods
Tooth Specimen Selection and Groups
The study protocol was approved by the University of Toronto Research Ethics Board (protocol reference #29482). One hundred fifty permanent mandibular first molars with 2 moderately curved mesial canals (25˚–35˚) were selected. Teeth were imaged with cone-beam computed tomographic imaging (Kodak 9000; Care- stream Dental LLC, Atlanta, GA) set at 66 kV, 10 mA, 10.8-second exposure, and a slice thickness of 76 mm to obtain a pretreatment outline of the root canals. Twenty-four teeth with 2 independent patent mesial canals were selected for further study. These were decoronated slightly above the cementoenamel junction, disinfected in 0.5% chloramine T solution, and stored in distilled water at 4◦C.
Before instrumentation, teeth were mounted on a custom attachment and imaged using a micro-CT system (SkyScan 1174v2: Bruker-microCT, Kontich, Belgium) at 50 kV and 800 mA and an isotropic resolution of 19.6 mm. Scanning was performed through 180˚ rotation around the vertical axis with a rotation step of 1◦ using a 0.5-mm-thick aluminum filter. The acquired images were reconstructed into cross-sectional slices with NRecon v.1.6.9 software (Bruker-microCT) using standardized parameters for beam hardening (15%), ring artifact correction (5%), and similar contrast limits. The volume of interest was selected extending from the furcation level to the apex of the root, resulting in the acquisition of 700 to 900 transverse cross sections per tooth in a bitmap (BMP) format. Root canal length, volume, surface area, and dentin thickness from the level of the furcation to the apex of the root were recorded using CTAn v.1.14.4 software (Bruker-microCT, Kontich, Belgium). Sample size calculation indicated that 16 root canals per group were required to support analysis with 80% power and 5% level of significance.
Subsequently, 24 mesial roots (48 root canals) were matched to create 8 groups of 3 roots based on the 3-dimensional (3D) morphologic aspects of the mesial canals. One root from each group was randomly assigned to 1 of the 3 experimental groups (n = 16) according to the canal preparation systems (ie, PTG, PTU, or PTN). After checking the normality assumption (Shapiro-Wilk test), the degree of homogeneity (baseline) of the 3 groups with respect to canal length, volume, and surface area was confirmed using the 1-way analysis of variance test with a significance level of 5% (a = .05).
Root Canal Preparation
A single experienced operator performed all procedures. Canals were accessed and the coronal third flared with Gates-Glidden drills 2 and 3 (Dentsply Maillefer). Apical patency was confirmed with a #10 K-type file (Dentsply Maillefer) passed through the apical foramen before and after canal preparation. The working length (WL) was determined by passing a #10 K-type file through the major foramen and withdrawing it 0.5 mm. A glide path was created using a ProGlider instrument (16/02) (Dentsply Maillefer) carried to the WL. All instruments used were taken to the WL in a continuous clockwise rotation generated by a 6:1 angle handpiece (Sirona, Bensheim, Germany) powered by an electric motor (VDW Silver Motor; VDW GmbH, Munich, Germany) at 300 rpm and 2.5 Ncm. The instrument sequence in the PTU and PTG groups was S1 (17/02), S2 (20/04), F1 (20/07), and F2 (25/08). In the PTN group, the sequence was X1 (17/04) and X2 (25/06). After 3 gentle in-and-out motion strokes in an apical direction, the instrument was removed from the canal and cleaned. This was repeated until the WL was reached, and then the instrument was discarded. After each step, the canal was irrigated with 20 mL 2.5% NaOCl using a disposable syringe fitted with a 30-G NaviTip needle (Ultradent, South Jordan, UT) placed 1 mm short of the WL. A final rinse with 5 mL 17% EDTA was followed by a 5-mL rinse with distilled water. Canals were dried with paper points (Dentsply Maillefer), imaged with a micro-CT system, and reconstructed with the same parameters used in pretreatment scans.
Outcome Measures
Color-coded 3D models of the root canals, pre- and post-preparation, were coregistered using automated image registration. Custom combinations of rigid to affine modules were used based on image intensity similarities (3D Slicer 4.3.1 software, available from http://www.slicer.org) with accuracy greater than 1 voxel. Unprepared (green) and prepared (red) matched canals were qualitatively compared using CTVol v.2.2.1 software (Bruker-microCT). The area of untouched canal surface was determined by calculating the number of static voxels (voxels present in the same position on the canal surface before and after instrumentation). The untouched area was expressed as a percentage of the total number of voxels present on the canal surface.
CTAn v.1.14.4 software was used to measure volume (in mm3), surface area (in mm2), structure model index (SMI), area (in mm2), perimeter (in mm), form factor, roundness, major diameter (in mm), minor diameter (in mm), and aspect ratio of the root canals before and after preparation. 3D evaluation was performed for the full canal length, and 2-dimensional (2D) evaluation was done for the apical 5 mm of the canal in 250 cross-sectional images per canal. Comparison parameters were calculated by subtracting values obtained for treated canals with those obtained from untreated counterparts. Criteria used for the calculation of the parameters have been published previously.
Canal transportation was assessed from a center of gravity calculated for each slice and connected along the z-axis with a fitted line through a total of 8583 cross sections in the PTU group, 8345 in the PTN group, and 8477 in the PTG group using XLSTAT-3DPlot for Windows (Addinsoft, New York, NY). The mean transportation (mm) was calculated by comparing the centers of gravity before and after treatment for the coronal, middle, and apical thirds of the canals.
The mean percentage decrease of dentin wall thickness was acquired by the superimposition of the data sets before and after canal preparation from the midpoint between the canal orifice and the foramen. Fifteen measurements of the width of dentin toward the distal aspect of the external root surface, perpendicular to a line connecting the centers of gravity, spaced by 1◦ in either the mesiobuccal or mesiolingual canals were recorded. Color-coded 3D models of dentin thick- ness throughout the root were created by CTAn v.1.14.4 software.
The Shapiro-Wilk test was used to assess the normality of the data. Results of untouched canal wall surface, volume, surface area, SMI, area, perimeter, roundness, form factor, major and minor diameters, and aspect ratio were compared between groups using the Kruskal-Wallis post hoc Dunn test and presented as median values or an interquartile range (IQR). Canal transportation and dentin wall thickness data were normally distributed and compared between groups with the 1-way analysis of variance post hoc Tukey test. Commercially available software (SPSS v17.0; SPSS Inc, Chicago, IL) was used for analysis at a 5% significance level.
Results
The median and IQR of static voxels indicating an untouched canal surface in each group are shown in Figure 1. A wide range in calculated percentages (0%–34%) was noted among specimens within groups; however, the analysis of the values recorded indicated that for most specimens the variance ranged from 6%–13%. Overall, the PTN group showed significantly higher (P < .05) median percentage values of static voxels (11.66%, IQR = 11.94) when compared with the PTG (3.57%, IQR = 9.92) and PTU (2.66%, IQR = 7.83) groups. No significant difference was noted between PTG and PTU.
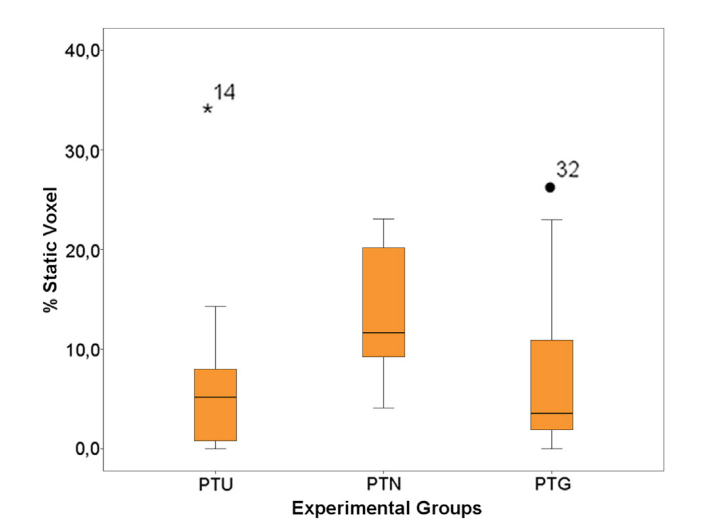
The results of the 2D and 3D analyses are shown in Tables 1 and 2, respectively. Preparation significantly increased all measured parameters in each group. Overall, the percentage increase in the surface area, the perimeter, and the minor diameter of the canals were significantly higher in the PTG and PTU groups than in the PTN group (P < .05). No statistical difference in form factor, roundness, major diameter, aspect ratio, or SMI was present among groups (P > .05). The PTU group produced a significantly larger increase in canal volume and surface area than the PTG and PTN groups in the coronal and middle thirds of canals (P < .05), but no significant differences were observed in the apical third. No significant difference in SMI was observed between groups (P > .05).
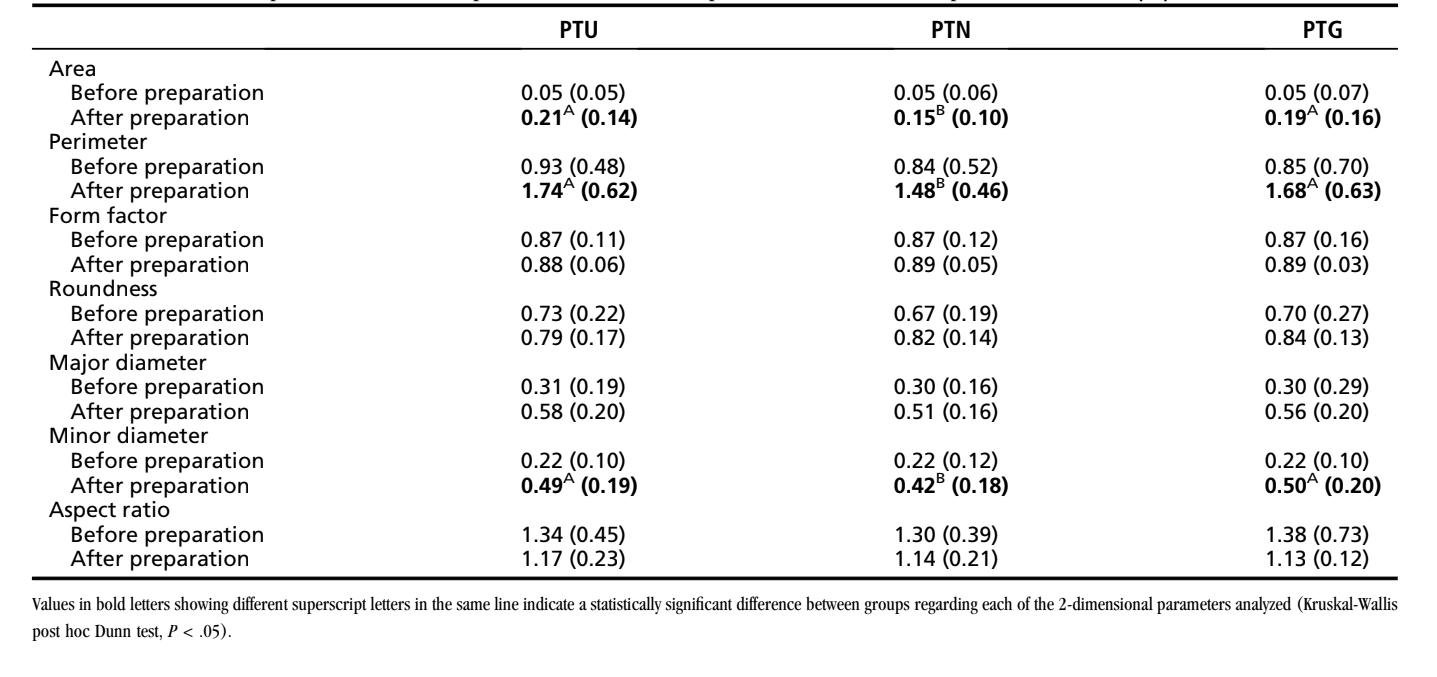
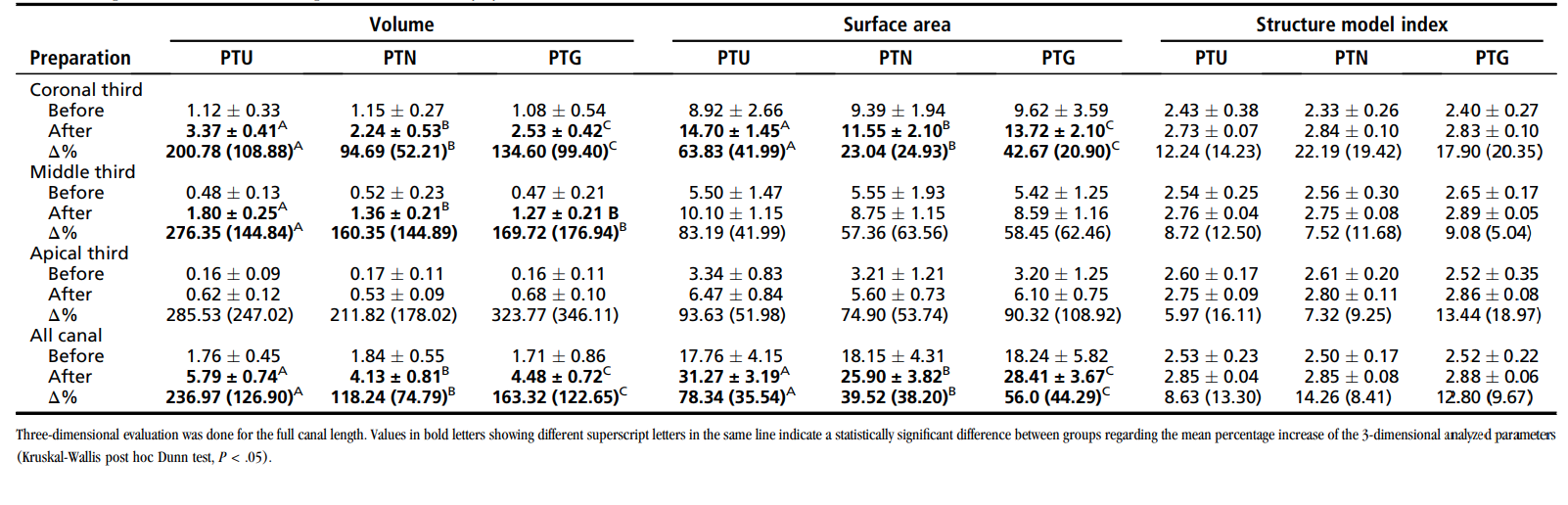
Preoperatively, canal cross sections were oval shaped (mean aspect ratio of 1.45), and the canal geometry was irregularly tapered (Fig. 2A). After preparation, the geometry of the canals was larger and showed a smooth taper in all groups (Fig. 2B). Changes in the canal shape, displayed as superimpositions of unprepared (green) and prepared (red) areas, showed that all groups maintained the overall canal shape (Fig. 2C and D).
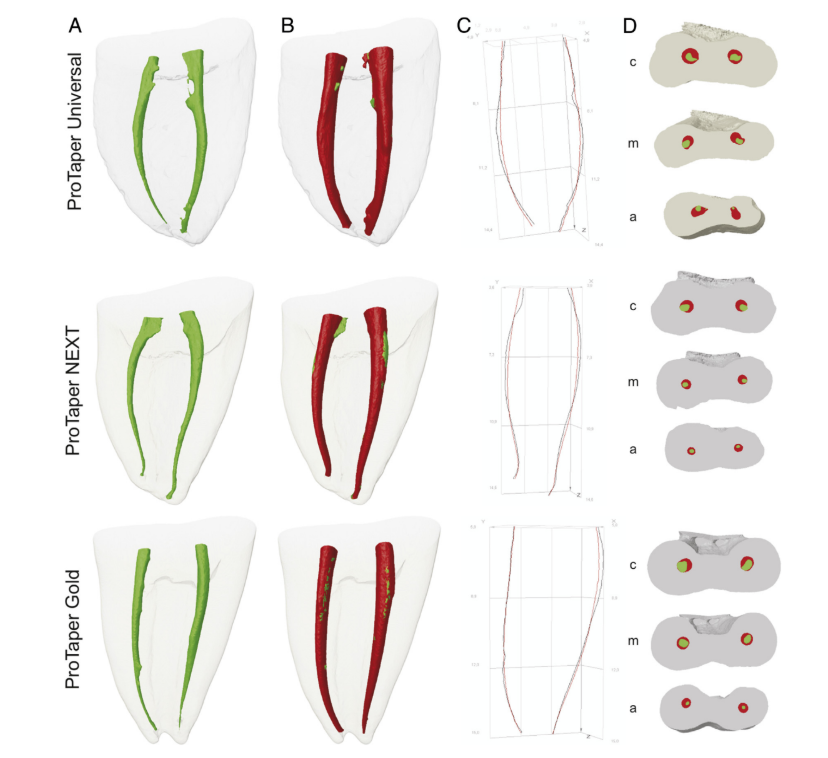
The results of canal transportation are summarized in Table 3 and graphically represented in Figure 2C and D. The highest transportation values were observed in the middle and apical thirds of the PTU group (~0.50 mm). Overall, the PTN (0.09 0.05 mm) and PTG (0.11 0.05 mm) groups had significantly less (P < .05) transportation than the PTU group (0.14 0.07 mm).
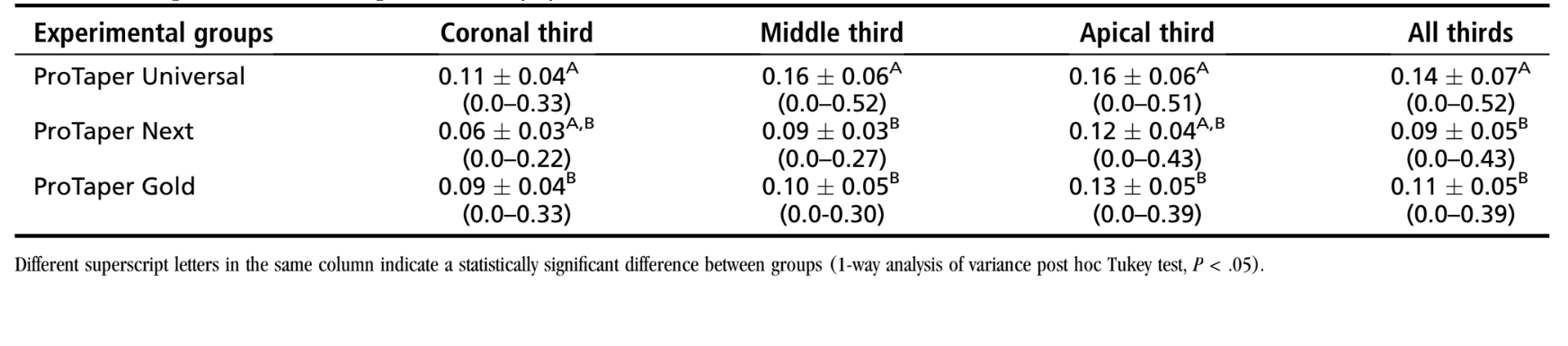
In the middle third of the root, the mean dentin thickness before preparation was 1.15 0.18 mm, 1.06 0.20 mm, and 1.10 0.32 mm in the PTU, PTN, and PTG groups, respectively. After preparation, dentin thickness ranged from 0.62 to 1.75 mm, 0.72 to 1.38 mm, and 0.72 to 1.83 mm in the PTU, PTN, and PTG groups, respectively. The percentage decrease in dentin thickness was significantly greater (P < .05) in the PTU group (29.93% ± 6.24%) compared with the PTN (17.71% ± 5.93%) and PTG (22.67% 2.96%) groups. Values for the PTN and PTG groups did not differ significantly (P > .05). Figure 3 shows a color-coded representation of the dentin thickness throughout the root of a representative specimen from each group. Thick structures are indicated in blue and green, whereas red indicates areas of thin dentin.
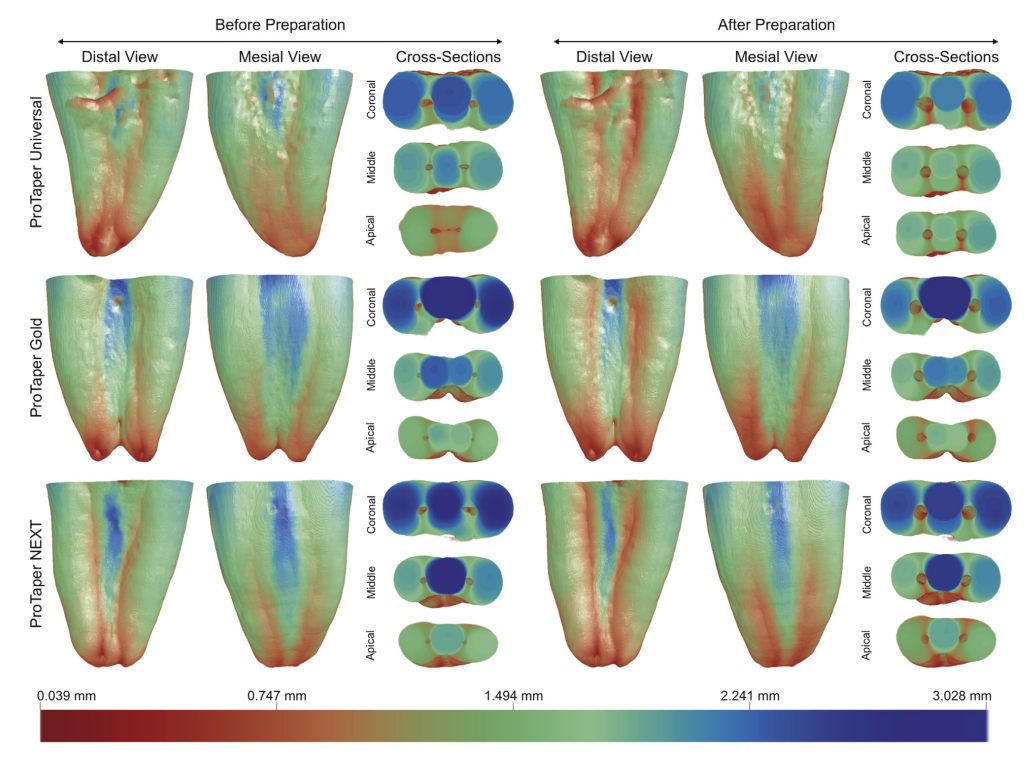
Discussion
Multirooted teeth have complex anatomy and present a greater challenge to successful endodontic therapy than single-rooted teeth. Continued evolution of instruments is intended to facilitate the task. This study evaluated the canal shaping characteristics of the newly introduced PTG system in comparison with those of the widely used PTN and PTU systems using micro-CT imaging, a nondestructive, reproducible, and well-established method for the 3D assessment of the root canal preparation. Unfortunately, the results with the PTG system cannot be compared with others because currently similar studies are not available.
All instruments showed untouched areas of the root canal wall, indicating that none were able to completely clean the dentin walls, which is in agreement with previous studies on NiTi rotary systems; however, it deserves attention that the mean range of the untouched areas of the root canal wall (6%–13%) were lower than previous reports using similar methodology. It has been shown that variations in canal geometry before instrumentation may have a greater effect on observed changes than the instrumentation techniques themselves. In this way, a less complex preoperative configuration of the root canals selected in this study may explain the results. Overall, the PTU and PTG systems resulted in significantly less untouched canal walls and a higher increase in the surface area, perimeter, and minor diameter of the canals than the PTN system. These results might be explained by differences in the design of the instruments. Although PTU and PTG share a similar geometry, the smaller dimensions, off-centered mass, and regressive taper of the PTN instruments should reduce the contact area with the canal and, therefore, its cutting ability.
Rotary NiTi instruments have been shown to maintain the original canal curvature well, even in extremely curved canals. In the current study, movements of the centers of gravity were metrically evaluated in absolute numbers (mm), slice by slice, as canal transportation. Overall, PTG and PTN produced significantly less canal transportation than PTU instruments. Despite PTU and PTG sharing geometric designs, they are manufactured from different alloys and the more flexible alloy of the PTG, enhanced through a proprietary heat treatment technology, imparts a reduced restoring force and may explain why these instruments remained more centered in the canal than PTU during use. This finding is supported by previous studies that compared transportation by M-wire systems with those made of conventional NiTi. Interestingly, although PTG and PTN share neither geometric design nor metallurgy, the differences did not significantly affect their centering ability. One explanation may be the improved flexibility of the PTN instruments as a consequence of its design features (off-centered mass of rotation and rectangular cross-section), alloy (M-wire), and the smaller dimensions of the instrument (25/0.06). The present results are comparable with recent publications on PTN used to prepare curved canals of extracted mandibular first molars.
Evaluation of dentin thickness is important because excess removal of dentin could predispose teeth to root fracture. Therefore, when an instrument remains centered in the canal, it is expected that more dentin is maintained, which may explain the greater percentage of remaining dentin thickness observed with PTG and PTN instruments. PTG and PTN systems also showed similar increases of volume and surface area in the coronal and middle thirds of the root canal despite their different dimensions. It could be hypothesized that heat treatment of the alloy in PTG instruments may predispose the instruments to plastic deformation and disruption of cutting edges during use, reducing its cutting ability. This finding corroborates previous literature that showed plastic deformation of instruments after clinical use as a result of the thermal pretreatment of the alloy. However, others have showed that PTN removed similar amounts of dentin as PTU. Interestingly, recent data on cutting efficiency of conventional and martensitic NiTi instruments showed the ‘‘softer’’ martensitic alloy was the most efficient instrument in lateral action. The authors hypothesized that the increased cutting efficiency of Hyflex CM1 (Coltene Whaledent, Cuyahoga Falls, OH) was related to the alloy’s thermomechanical processing and configuration of the flutes; however, in this study, acrylic blocks and bovine dentin were used as substrates, and the findings have not been corroborated by other studies. It is worth pointing out that, in addition to the prescribed manufacturers’ directions, coronal flaring was performed with Gates-Glidden drills. Therefore, changes of the analyzed parameters at this level should be interpreted with caution because they may not represent the efficacy of the preparation systems themselves but also the additional action of the burs. Considering that cutting ability of an endodontic instrument is a result of the complex interrelationship of parameters, this assumption warrants further investigation.
Teeth used in this study were anatomically matched in terms of preoperative geometric parameters determined by micro-CT imaging. This procedure creates a reliable baseline and ensures the comparability of the groups by standardization of the 3D canal morphology in each sample, enhancing internal validity and potentially eliminating significant anatomic biases that may confound the outcomes. Although significant differences regarding canal transportation and remaining dentin thickness were obtained, the clinical relevance of the results obtained remains questionable and may not be clinically significant or affect treatment outcomes. Hence, it is important for clinicians to have impartial information regarding the various characteristics that may affect the shaping characteristics of the PTU, PTN, and PTG systems to facilitate good choices to meet anatomic challenges.
Conclusions
Within the limitations of this study, PTG and PTN resulted in less transportation and greater ability to maintain dentin thickness than PTU. Differences in measured parameters were small and did not appear to influence the system’s ability to shape moderately curved root canals. Future research should be focused on comparing these systems in severely curved canals and examining PTG with systems manufactured from similar metallurgy.
Authors: Jason Gagliardi, Marco Aurelio Versiani, Manoel Damiao de Sousa-Neto, Andres Plazas-Garzon and Bettina Basrani
References:
- Kakehashi S, Stanley HR, Fizgerald RJ. The effects of surgical exposures on dental pulps in germ-free and conventional laboratory rats. J South Calif Dent Assoc 1966;34:449–51.
- Sjøgren U, Figdor D, Persson S, Sundqvist G. Influence of infection at the time of root filling on the outcome of endodontic treatment of teeth with apical periodontitis. Int Endod J 1997;30:297–306.
- Shuping GB, Ørstavik D, Sigurdsson A, Trope M. Reduction of intracanal bacteria using nickel-titanium rotary instrumentation and various medications. J Endod 2000;26:751–5.
- Glossen CR, Haller RH, Dove SB, del Rio CE. A comparison of root canal preparations using Ni-Ti hand, Ni-Ti engine-driven, and K-Flex endodontic instruments. J Endod 1995;21:146–51.
- Gao Y, Gutmann JL, Wilkinson K, et al. Evaluation of the impact of raw materials on the fatigue and mechanical properties of ProFile Vortex rotary instruments. J Endod 2012;38:398–401.
- Ye J, Gao Y. Metallurgical characterization of M-Wire nickel-titanium shape memory alloy used for endodontic rotary instruments during low-cycle fatigue. J Endod 2012;38:105–7.
- Arias A, Singh R, Peters OA. Torque and force induced by ProTaper Universal and ProTaper Next during shaping of large and small root canals in extracted teeth. J Endod 2014;40:973–6.
- Gergi R, Osta N, Bourbouze G, et al. Effects of three nickel titanium instrument systems on root canal geometry assessed by micro-computed tomography. Int Endod J 2015;48:162–70.
- Marceliano-Alves MF, Sousa-Neto MD, Fidel SR, et al. Shaping ability of single-file reciprocating and heat-treated multifile rotary systems: a micro-CT study. Int Endod J 2014 Nov 14. http://dx.doi.org/10.1111/iej.12412. [Epub ahead of print].
- Peters OA, Laib A, Göhring TN, et al. Changes in root canal geometry after preparation assessed by high-resolution computed tomography. J Endod 2001;27:1–6.
- Versiani MA, Pécora JD, Sousa-Neto MD. The anatomy of two-rooted mandibular canines determined using micro-computed tomography. Int Endod J 2011;44: 682–7.
- Bergmans L, Van Cleynenbreugel J, Wevers M, et al. A methodology for quantitative evaluation of root canal instrumentation using microcomputed tomography. Int Endod J 2001;34:390–8.
- Versiani MA, Leoni GB, Steier L, et al. Micro-computed tomography study of oval- shaped canals prepared with the self-adjusting file, Reciproc, WaveOne, and Pro-Taper universal systems. J Endod 2013;39:1060–6.
- Zhao D, Shen Y, Peng B, et al. Root canal preparation of mandibular molars with 3 nickel-titanium rotary instruments: a micro-computed tomographic study. J Endod 2014;40:1860–4.
- Çapar ID, Ertas H, Ok E, et al. Comparative study of different novel nickel-titanium rotary systems for root canal preparation in severely curved root canals. J Endod 2014;40:852–6.
- Peters OA, Schönenberger K, Laib A. Effects of four Ni-Ti preparation techniques on root canal geometry assessed by micro computed tomography. Int Endod J 2001;34: 221–30.
- Peters OA, Boessler C, Paqué F. Root canal preparation with a novel nickel-titanium instrument evaluated with micro-computed tomography: canal surface preparation over time. J Endod 2010;36:1068–72.
- Paqué F, Balmer M, Attin T, et al. Preparation of oval-shaped root canals in mandibular molars using nickel-titanium rotary instruments: a micro-computed tomography study. J Endod 2010;36:703–7.
- Bürklein S, Börjes L, Schäfer E. Comparison of preparation of curved root canals with Hyflex CM and Revo-S rotary nickel-titanium instruments. Int Endod J 2014; 47:470–6.
- Saber SE, Nagy MM, Schäfer E. Comparative evaluation of the shaping ability of WaveOne, Reciproc and OneShape single-file systems in severely curved root canals of extracted teeth. Int Endod J 2015;48:109–14.
- Bürklein S, Mathey D, Schäfer E. Shaping ability of ProTaper Next and BT-RaCe nickel-titanium instruments in severely curved root canals. Int Endod J 2015;48:774–81.
- Wu H, Peng C, Bai Y, et al. Shaping ability of ProTaper Universal, WaveOne and Pro-Taper Next in simulated L-shaped and S-shaped root canals. BMC Oral Health 2015; 15:27.
- Pérez-Higueras JJ, Arias A, de la Macorra JC, et al. Differences in cyclic fatigue resistance between ProTaper Next and ProTaper Universal instruments at different levels. J Endod 2014;40:1477–81.
- Hieawy A, Haapasalo M, Zhou H, et al. Phase transformation behavior and resistance to bending and cyclic fatigue of ProTaper gold and ProTaper universal instruments. J Endod 2015;41:1134–8.
- Pongione G, Pompa G, Milana V, et al. Flexibility and resistance to cyclic fatigue of endodontic instruments made with different nickel-titanium alloys: a comparative test. Ann Stomatol (Roma) 2012;3:119–22.
- Elnaghy AM, Elsaka SE. Evaluation of root canal transportation, centering ratio, and remaining dentin thickness associated with ProTaper Next instruments with and without glide path. J Endod 2014;40:2053–6.
- Saber SE, Nagy MM, Schäfer E. Comparative evaluation of the shaping ability of Pro-Taper Next, iRaCe and Hyflex CM rotary NiTi files in severely curved root canals. IntEndod J 2015;48:131–6.
- Kishen A. Mecanisms and risk factors for fracture predilection in endodontically treated teeth. Endod Topics 2006;13:57–83.
- Tang W, Wu Y, Smales RJ. Identifying and reducing risks for potential fractures in endodontically treated teeth. J Endod 2010;36:609–17.
- Hülsmann M, Peters OA, Dummer P. Mechanical preparation of root canals: shaping goals, techniques and means. Endod Topics 2005;10:30–76.
- Shen Y, Coil JM, Zhou H, et al. HyFlex nickel-titanium rotary instruments after clinical use: metallurgical properties. Int Endod J 2013;46:720–9.
- Morgental RD, Vier-Pelisser FV, Kopper PM, et al. Cutting efficency of conventional and martensitic nickel-titanium instruments for coronal flaring. J Endod 2013;39: 1634–8.
- Versiani MA, P´ecora JD, Sousa-Neto MD. Microcomputed tomography analysis of the root canal morphology of single-rooted mandibular canines. Int Endod J 2013;46: 800–7.