Root dentinal microcracks: a post-extraction experimental phenomenon?
Abstract
Aim: To investigate the prevalence, location and pattern of pre-existing microcracks in non-endodontically treated teeth from fresh cadavers. Micro-computed tomography (micro-CT) technology was used as the analytical tool enabling full screening of the root dentine with the teeth retained in their original alveolar socket.
Methodology: As a pilot study and to validate the present method, a series of 4 high-resolution scans were performed on one bone-block specimen with teeth collected post-mortem: (i) entire bone-block including the teeth, (ii) second molar tooth extracted atraumatically from the bone-block, (iii) extracted tooth dehydrated to induce dentinal defects and (iv) entire bone-block following reinsertion of the extracted tooth into its matching alveolar socket. In the main study, forty-two dentoalveolar maxillary and mandibular bone-blocks each containing 3–5 adjacent teeth (a total of 178 teeth) were collected post-mortem and scanned in a micro-CT device. All cross-section images of the 178 teeth (n = 65 530) were screened from the cementoenamel junction to the apex to identify the presence of dentinal defects.
Results: In the pilot study, the microcracks observable when the dehydrated tooth was outside the bone-block remained detectable when the entire bone-block plus reinserted tooth was scanned. This means that the screening process revealed the presence of the same microcracks in both experimental situations (the tooth outside and inside the maxillary bone-block). From a total of 178 teeth in the bone-blocks removed from cadavers, 65 530 cross-sectional images were analysed and no dentinal microcracks were detected.
Conclusions: This in situ cadaveric model revealed the lack of pre-existing dentinal microcracks in non-endodontically treated teeth. Thus, the finding of dentinal microcracks observed in previous cross-sectional images of stored extracted teeth is unsound and not valid. It should be assumed that microcracks observed in stored extracted teeth subjected to root canal procedures are a result of the extraction process and/or the post-extraction storage conditions. Therefore, as a consequence, the presence of such dentinal microcracks in stored extracted teeth – observable in cross-sectional images of the roots – should be referred to as experimental dentinal microcracks.
Introduction
During the investigation of vertical root fractures (VRFs), the microstructural integrity of root dentine and cementum has been evaluated using destructive (tooth-sectioning) (Hin et al. 2013, Liu et al. 2013, (tooth-sectioning) (Hin et al. 2013, Liu et al. 2013, Arias et al. 2014, Ashwinkumar et al. 2014, Karataş et al. 2016, Saber & Schäfer 2016, Bahrami et al. 2017, Kfir et al. 2017) and non-destructive (micro-computed tomography [micro-CT]) experimental models (De-Deus et al. 2014, 2015, 2016, 2017a,b, Bayram et al. 2017, Pradeep Kumar et al. 2017, Zuolo et al. 2017). Most of these studies used either teeth that had been stored for varying periods of time (Hin et al. 2013, Liu et al. 2013, De-Deus et al. 2014, 2015, 2016, 2017a, Karataş et al. 2016, Bayram et al. 2017, Zuolo et al. 2017) or were freshly extracted (Ashwinkumar et al. 2014, Saber & Schäfer 2016, Kfir et al. 2017, PradeepKumar et al. 2017) with only a few studies being conducted using cadaveric models (Arias et al. 2014, Bahrami et al. 2017, De-Deus et al. 2017b).
The use of a non-destructive high-resolution imaging technology, that is micro-CT, has made it possible to gain a more reliable insight into the phenomenon of dentinal microcrack formation. Micro-CT allows the internal structure of opaque objects (e.g. teeth) to be observed by screening hundreds of slices per specimen, where the full extent of crack lines can be mapped (De-Deus et al. 2014, 2015, 2016, 2017a,b, Bayram et al. 2017, PradeepKumar et al. 2017, Zuolo et al. 2017). The use of micro-CT thus allows root dentine and cementum to be observed in their original state, that is after extraction, and then examined again after root canal procedures. Based on this method, two main conclusions have been drawn: (i) the lack of relationship between dentinal microcrack formation and mechanical preparation of root canals with nickel–titanium (NiTi) instruments per se (De-Deus et al. 2014, 2015, 2016, 2017a,b, Bayram et al. 2017, Zuolo et al. 2017) and (ii) the recognition of pre-existing microcracks as a phenomenon in untreated teeth (De-Deus et al. 2014, 2015, 2016, 2017a,b, Bayram et al. 2017, PradeepKumar et al. 2017, Zuolo et al. 2017). Pre-existing microcracks are microstructural defects in roots of non-endodontically treated teeth with their aetiology being credited to factors such as age, parafunctional stresses (Yang et al. 1995, Chan et al. 1998) or restorative procedures (Kishen 2006, Shemesh et al. 2009).
Although rarely reported in destructive sectioning studies, pre-existing microcracks have been observed in non-endodontically treated specimens even in the initial studies that focused mostly on the relationship between the development of dentinal defects and root canal preparation techniques (Arias et al. 2014, Karataş et al. 2016, Bahrami et al. 2017, Kfir et al. 2017). Interestingly, the use of micro-CT technology in studies using stored teeth revealed a high incidence (ranging from 12.31% to 41.44%) of pre-existing microcracks in the baseline images acquired from untreated teeth (De-Deus et al. 2014, 2015, 2016, 2017a, Bayram et al. 2017, Zuolo et al. 2017). Actually, information provided by untreated control groups has been significant and controversial. Most often, no microcracks can be observed when stored sound teeth are sectioned horizontally (Shemesh et al. 2009, Karataş et al. 2016, Kfir et al. 2017), while in some studies using cadaveric models microcracks have been reported in the untreated control groups (Arias et al. 2014, Bahrami et al. 2017). Conversely, a low prevalence of pre-existing microcracks was reported when evaluating freshly extracted teeth (7.1%) (PradeepKumar et al. 2017) or in a cadaveric model (2.46%) (De-Deus et al. 2017b) when using micro-CT technology. This means that the phenomenon of pre-existing microcracks must be reconsidered as a consequence of the new evidence provided by the micro-CT imaging method and the use of either freshly extracted teeth (De-Deus et al. 2017b, PradeepKumar et al. 2017) or teeth within a cadaver model (De-Deus et al. 2017b).
In summary, the existence of pre-existing microcracks has been controversial. The somewhat puzzling occurrence of pre-existing microcracks has created interest in potential aetiological factors as well as in determining whether VRFs are preceded by such microstructural defects. Considering its as-yet-unknown aetiology as well as the lack of knowledge on this phenomenon, the current study aimed to investigate the prevalence, location and pattern of pre-existing microcracks in non-endodontically treated teeth from fresh cadavers. Micro-CT technology was used as the analytical tool enabling full screening of the root dentine with the teeth retained in their original alveolar socket. The core hypothesis being tested was that pre-existing microcracks occur at a high frequency in non-endodontically treated teeth.
Materials and methods
Sample selection
Forty-two dentoalveolar maxillary and mandibular bone-blocks, each containing 3–5 adjacent teeth (a total of 178 teeth), were collected post-mortem during the autopsy of several adult donors. The family members gave their informed consent which was obtained under a research protocol approved by the local Forensic Department and the National Committee on Health Research Ethics (protocol no. 931.732). The age of the donors ranged from 19 to 44 years (mean age, 31 years). Inclusion criteria were the presence of non-carious maxillary or mandibular first and second pre-molars and molars surrounded by alveolar bone and periodontal ligament. Bone-blocks with teeth were stored at —20 °C and submitted to the experimental procedures within 40 days from their collection.
Before the scanning procedures, frozen bone-blocks were removed from the freezer and placed into a refrigerator at a constant temperature of 8°C for a slow defrost. After 3–4 h, each bone-block was scanned in a micro-CT device (SkyScan 1173; Bruker-microCT, Kontich, Belgium) using an isotropic resolution of 13.18 lm at 90 kV and 88 mA through 360° rotation around the vertical axis, with a rotation step of 0.5°, camera exposure time of 1000 ms and frame averaging of 5. The X-rays were filtered with a 1-mm-thick aluminium filter. The acquired images were reconstructed into cross-sectional slices with NRecon v.1.6.10 software (Bruker-microCT) using standardized parameters for beam hardening (15%), ring artefact correction and contrast limits (0.0095–0.03), resulting in the acquisition of 1300– 1600 transverse cross-sections per bone-block.
Pilot study – validation method
Validation of the present method was based on 4 high-resolution micro-CT scans of a single bone-block containing 3 teeth (one premolar, one-first molar and one-second molar) following the same parameters previously described. The sequence of micro-CT scans was as follows: (i) entire bone-block, (ii) extracted tooth, (iii) dehydrated extracted tooth and (iv) entire bone-block after reinsertion of the extracted tooth into its alveolar socket (Fig. 1). The integrity of the dentine (the presence of dentinal microcracks) was evaluated by screening the cross-sectional images obtained in the reconstruction step, from the cementoenamel junction to the root apex, by 3 blinded calibrated examiners. The calibration process was based on viewing sessions using cross-sectioning images with previously identified microcracks. The image analysis was repeated twice at 2-week intervals to validate the process of microcracks identification.
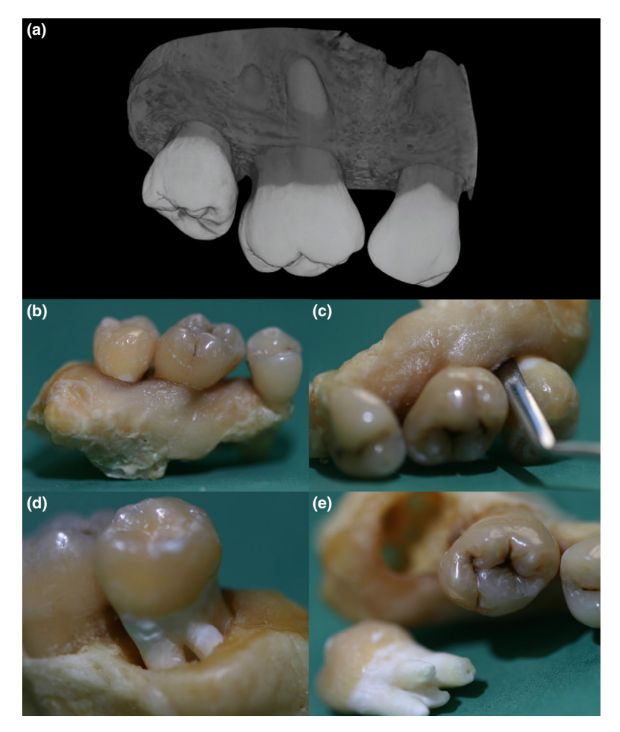
In the first scan, no microcracks were observed (Figs 2a,b and 3a,b). Then, the maxillary second molar tooth was atraumatically removed from the bone-block by avoiding touching or damaging the surrounding tissues (Fig. 1c,d and e). This technique involved a careful detachment of 2/3 of the roots with periotomes until luxation occurred and, to minimize potential tooth damage, extraction forces were used only for tooth withdrawal, and not to loosen it. The extracted molar was immediately scanned, and the cross-sectional images were screened as described above. No microcracks were observed in the second scan (Figs 2c and 3c).
Aiming to induce the development of dentinal defects, the second molar tooth was submitted to a dehydration process using a standard graded series of alcohols (50%, 60%, 70%, 80%, 90% and 100% ethanol). Then, the tooth was placed into an auto-desiccator cabinet (Bel-Art automatic desiccator clear 2.0, Wayne, NJ, USA) and scanned on a weekly-basis to verify the presence of microcracks. After a period of 3 months, the scan (third scan) clearly revealed the presence of dentinal microcracks (Figs 2d and 3d). Subsequently, the specimen was carefully reinserted in its original alveolar socket and the entire bone-block was rescanned (fourth scan). The image analysis of the cross-sections revealed that the microcracks observed when the tooth was outside the bone-block remained detectable when the entire bone-block was scanned (Figs 2e,f and 3e,f).
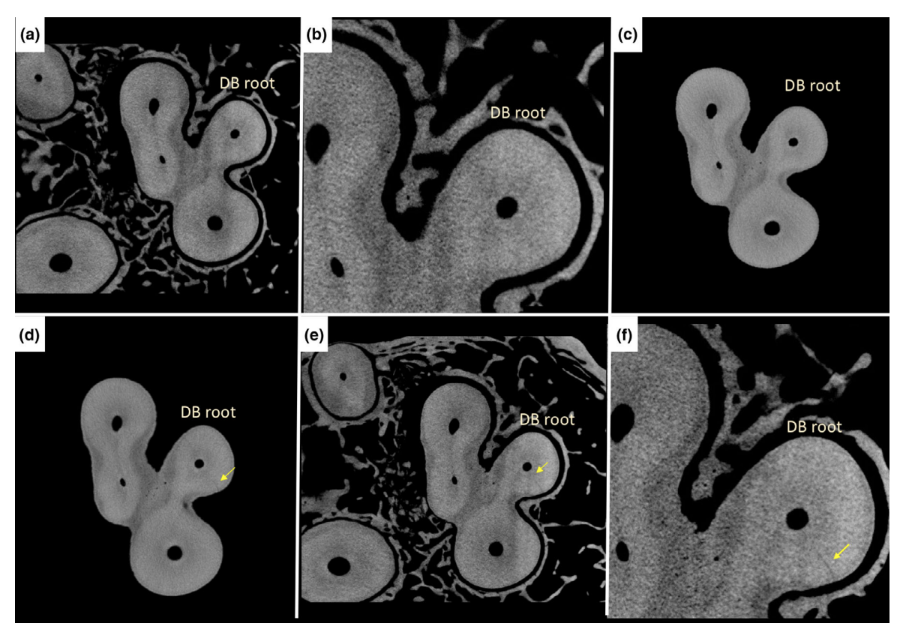
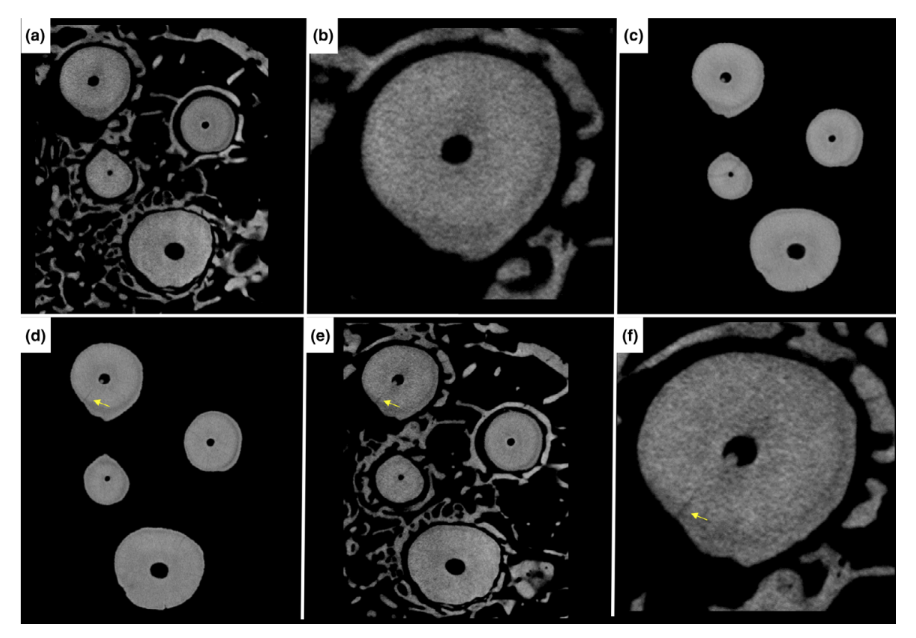
Image analysis
Visualization and qualitative analysis of the reconstructed image stacks of the 42 bone-blocks were assessed using CTVol v.2.3 software (Bruker-microCT). All cross-sectional images of the 178 teeth (n = 65 530) were screened from the cementoenamel junction to the apex to identify the presence of dentinal defects. Three previously calibrated examiners, blinded to the experimental design, screened all images at 2-week intervals. In case of divergence, images were evaluated together until full agreement was reached (De-Deus et al. 2016).
Results
In the pilot study, microcracks observed when the tooth was outside the bone-block remained detectable when the entire maxillary segment was scanned, which validated the method of assessing dentinal microcracks in a fresh cadaver model through micro-CT technology (Figs 1–3).
From a total of 178 teeth in the bone-blocks removed from cadavers, 65 530 cross-section images were analysed and no dentinal microcracks were detected. Figs 4 and 5 show representative images from coronal, middle and apical thirds of a selection of teeth evaluated in the study.
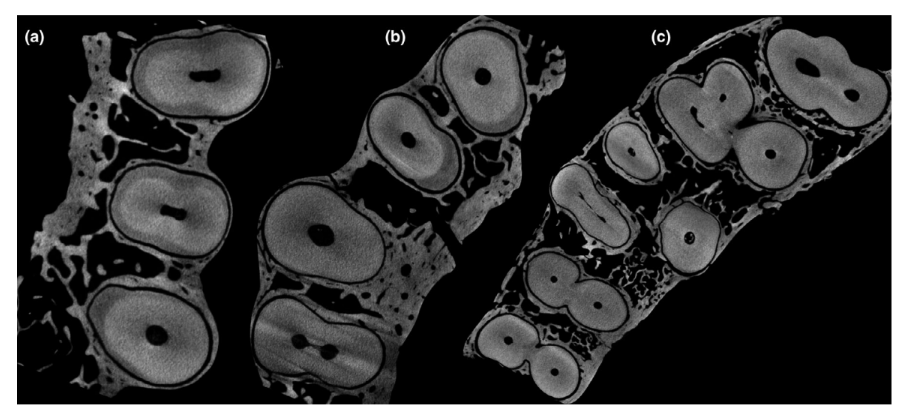
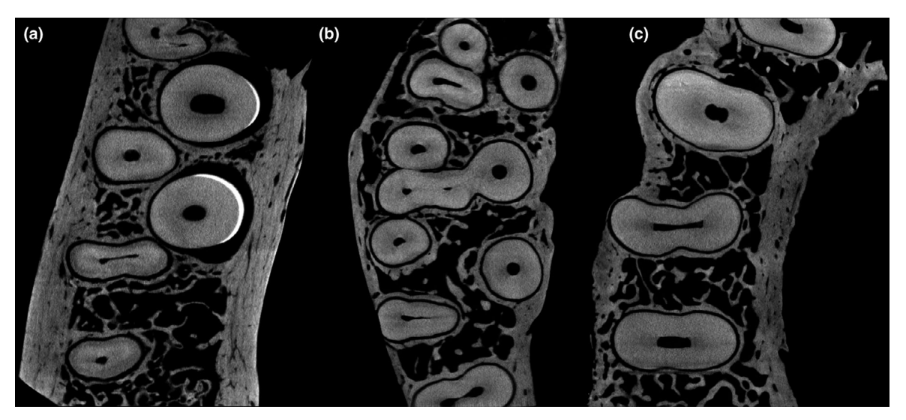
Discussion
In the current study, the incidence of dentinal microcracks in non-endodontically treated teeth was assessed in situ through micro-CT images of 178 teeth in maxillary and mandibular bone-blocks obtained from 42 fresh cadavers. No pre-existing dentinal microcrack was observed, refuting the core hypothesis. The absence of such dentinal microcracks in a methodology that is under close to in vivo conditions – a human cadaver model – suggests that microcracks may occur due to post-extraction manipulation or the storage conditions of the experimental teeth. This finding means that such dentinal microcracks – observable in cross-sectional images of the roots – may not exist in the clinical setting; in fact, thus far this type of dentinal defect has been observable only under post-extraction experimental conditions (De-Deus et al. 2014, 2015, 2016, 2017a, Bayram et al. 2017, PradeepKumar et al. 2017, Zuolo et al. 2017).
The present result contrasts with the accumulated knowledge regarding dentinal microcrack formation that has been published since 2009 (De-Deus et al. 2014, 2015, 2016, 2017a, Bayram et al. 2017, PradeepKumar et al. 2017, Zuolo et al. 2017). Actually, the concept that dentinal microcracks is a post-extraction experimental phenomenon are partially supported by recent insights on this topic. Shemesh et al. (2018) reported the impact of environmental conditions on dentinal tissue and demonstrated that loss of water produces stresses that are sufficient to induce spontaneous dentinal defects, demonstrating experimentally that the biomechanical response of root dentine is highly influenced by its degree of hydration. This is in accordance with previous findings that showed that residual microstrain concentrations in hydrated roots was a controlled phenomenon and also that dehydrated dentine had lower toughness (Jameson et al. 1993, Kahler et al. 2003,. Kruzic et al. 2003) and was more brittle (Huang et al. 1992). Thus, results reported in the study of Adorno et al. (2013) may be thought of as a consequence of tooth dehydration, since microcrack propagation continued in root slices even after 1 month of storage, even though no further stress was applied to the dentine. In this sense, the dehydration process that teeth endure outside of the oral environment may explain the high prevalence (12.31% to 41.44%) of dentinal microcracks in the baseline images of non-endodontically treated stored teeth evaluated through micro-CT technology (De-Deus et al. 2014, 2015, 2016, 2017a, Bayram et al. 2017, Zuolo et al. 2017), considering that the extracted teeth were obtained from tooth banks that used a variety of storage conditions.
Another important study used micro-CT to evaluate the prevalence, location and pattern of pre-existing dentinal microcracks in 633 freshly extracted non-endodontically treated teeth and found dentinal defects in 45 teeth (7.1% of the sample) (PradeepKumar et al. 2017). Similarly, De-Deus et al. (2017b) using a cadaveric model also reported 2.46% of dentinal defects in the baseline images from non-endodontically treated teeth. In accordance with the present results, these important outcomes establish a more realistic prevalence of pre-existing dentinal microcracks in extracted teeth, in contrast with the substantial number of defects reported in previous micro-CT studies using stored teeth (De-Deus et al. 2014, 2015, 2016, 2017a, Bayram et al. 2017, Zuolo et al. 2017). These findings emphasize the low prevalence of pre-existing microcracks and raise serious doubts about the validity of most studies on dentinal cracks using extracted teeth, since cracks are likely to be a consequence of the post-extraction experimental conditions. Based on this scientific evidence, it may be inferred that dehydration of dentinal tissue is the main cause of microcracks in non-endodontically treated teeth reported over the last decade. The prevalence of this phenomenon is thus a function of the interplay between the origin of the specimen – storage versus freshly extracted teeth/cadaveric model, and the analytical method used – sectioning versus non-destructive micro-CT. In a broader sense, the development of VRFs in endodontically treated teeth is usually attributed to factors such as age, the root and root canal anatomy, masticatory function and/or the presence of excursive interferences or parafunctions that teeth could be subjected to during a patients’ life (Arias et al. 2014).
However, it is possible that VRFs develop as a consequence of cracked or split teeth initiated originally from the crown. Hence, it is important to consider the possible implications of the conditions under which teeth are stored when analysing the results of laboratory studies since unintentional dehydration will introduce systematic experimental flaws, irrespective of care taken during the rest of the experiment. This strongly suggests that in situ approaches such as models using fresh cadavers should be considered as a gold standard to assess the behaviour of dentinal tissue in terms of crack initiation and propagation.
The methodology used in the current study appears to be the close to an ideal experimental model to study the phenomenon of microcracks and the overall status of the dentine. The use of an in situ fresh cadaveric model, in which the bone and periodontal ligament remained preserved and also, the viscoelastic properties of the attachment apparatus, together with a highly accurate and non-destructive imaging method (micro-CT) for the assessment of the integrity of dentinal tissue has clear advantages over other methodologies used previously in the study of dentinal defects, that is sectioning and micro-CT analysis of stored teeth. Furthermore, the cadaveric model avoids the impact of tooth extractions and thus the use of periotomes, luxators or forceps, which usually are suggested as generators of dentinal defects. However, it is necessary to emphasize that the sampling used in the current study has one limitation, the age range of the cadavers which was between 19 and 44 years old (mean age, 31 years). Therefore, future work should focus on assessing the presence of dentinal defects in older cadavers.
As stated in the first study on dentinal microcracks using a cadaveric model and micro-CT (De-Deus et al. 2017b), there is no international agreement, general regulations or standards of tissue banking on a specific storage temperature for teeth inside bone-blocks. A statement from the American Association of Tissue Banks (2008) recommended a storage temperature of —20 °C for up to 6 months and —40 °C for longer periods of deep-frozen preservation. However, the influence of storage time and freezing temperatures on the biomechanical properties of teeth are not entirely understood and are still to be determined. In the present study, the storage temperature of the cadaveric bone-blocks followed that used by De-Deus et al. (2017b) and did not affect the structure of the bone or teeth, which was —20 °C, as recommended, with a period of slow defrost before scanning and further experimental procedures.
Concerns regarding whether the scanning resolution of micro-CT images may or may not be sufficient to detect smaller microcracks have also been raised (Pop et al. 2015, De-Deus et al. 2016, PradeepKumar et al. 2017). Nevertheless, the validation of the micro-CT imaging method for the observation of the dentinal defects in extracted teeth has already been reported (De-Deus et al. 2016); it was demonstrated that defects visualized by direct observation of dentine with reflected light microscopy (using the sectioning method) are also visualized in the reconstructed cross-sectional images obtained by high-resolution micro-CT scans. However, the same is not necessarily valid when the teeth are scanned within cadaveric bone-blocks. Therefore, due to the innovative character of the observation of the dentinal defects in the images scanned from cadaveric bone-blocks, the validation of the method was necessary to eliminate any possibility of false-negative results. The results revealed that the screening process was able to demonstrate the presence of the same microcracks in both experimental setups (tooth outside and inside the maxillary bone-block), validating the method to assess dentinal microcracks in a fresh cadaver model through micro-CT technology.
The result of this study suggests that future works should focus on the existence of dentinal root dentinal microcracks in non-endodontically treated teeth. In the meantime, until proven otherwise, it should be assumed that dentinal microcracks observed in stored extracted teeth subjected to root canal procedures are in fact a result of the extraction process and/or the post-extraction storage conditions. As a consequence, the presence of such dentinal microcracks in stored extracted teeth – observable in cross-sectional images of the roots under experimental conditions – should be referred to as experimental dentinal microcracks.
Conclusions
This in situ cadaveric model revealed the absence of pre-existing dentinal microcracks in non-endodontically treated teeth. This means that the prevalence of dentinal microcracks observed in previous cross-sectional images of stored extracted teeth is flawed. It also questions whether microcracks – observable in cross-sectional images of roots in extracted teeth - really occur in non-endodontically treated teeth in the clinical setting.
Authors: G. De-Deus, D. M. Cavalcante, F. G. Belladonna , J. Carvalhal, E. M. Souza, R. T. Lopes, M. A. Versiani, E. J. N. L. Silva & P. M. H. Dummer
References
- Adorno CG, Yoshioka T, Jindan P, Kobayashi C, Suda H (2013) The effect of endodontic procedures on apical crack initiation and propagation ex vivo. International Endodontic Journal 46, 763–8.
- American Association of Tissue Banks (2008) AATB Standards for Tissue Banking (Section E: E4.120 Frozen and Cryopreserved Tissue), 12th edn. McLean, VA: AATB.
- Arias A, Lee YH, Peters CI, Gluskin AH, Peters OA (2014) Comparison of 2 canal preparation techniques in the induction of microcracks: a pilot study with cadaver mandibles. Journal of Endodontics 40, 982–5.
- Ashwinkumar V, Krithikadatta J, Surendran S, Velmurugan N (2014) Effect of reciprocating file motion on microcrack formation in root canals: an SEM study. International Endodontic Journal 47, 622–7.
- Bahrami P, Scott R, Galicia JC, Arias A, Peters OA (2017) Detecting dentinal microcracks using different preparation techniques: an in situ study with cadaver mandibles. Journal of Endodontics 43, 2070–3.
- Bayram HM, Bayram E, Ocak M, Uzuner MB, Geneci F, Celik HH (2017) Micro-computed tomographic evaluation of dentinal microcrack formation after using new heat-treated nickel-titanium systems. Journal of Endodontics 43, 1736–9.
- Chan CP, Tseng SC, Lin CP, Huang CC, Tsai TP, Chen CC (1998) Vertical root fracture in nonendodontically treated teeth - a clinical report of 64 cases in Chinese patients. Journal of Endodontics 24, 678–81.
- De-Deus G, Silva EJ, Marins J et al. (2014) Lack of causal relationship between dentinal microcracks and root canal preparation with reciprocation systems. Journal of Endodontics 40, 1447–50.
- De-Deus G, Belladonna FG, Souza EM et al. (2015) Micro-computed tomographic assessment on the effect of ProTaper Next and Twisted File Adaptive systems on dentinal cracks. Journal of Endodontics 41, 1116–9.
- De-Deus G, Belladonna FG, Marins JR et al. (2016) On the causality between dentinal defects and root canal preparation: a micro-CT assessment. Brazilian Dental Journal 27, 664–9.
- De-Deus G, Belladonna FG, Silva EJNL et al. (2017a) Micro-CT assessment of dentinal micro-cracks after root canal filling procedures. International Endodontic Journal 50, 895–901.
- De-Deus G, Carvalhal JCA, Belladonna FG et al. (2017b) Dentinal microcrack development after canal preparation: a longitudinal in situ micro-computed tomography study using a cadaver model. Journal of Endodontics 43, 1553–8.
- Hin ES, Wu MK, Wesselink PR, Shemesh H (2013) Effects of Self-Adjusting File, Mtwo, and ProTaper on the root canal wall. Journal of Endodontics 39, 262–4.
- Huang TJ, Schilder H, Nathanson D (1992) Effects of moisture content and endodontic treatment on some mechanical properties of human dentin. Journal of Endodontics 18, 209–15.
- Jameson MW, Hood JA, Tidmarsh BG (1993) The effects of dehydration and rehydration on some mechanical properties of human dentine. Journal of Biomechanics 26, 1055– 65.
- Kahler B, Swain MV, Moule A (2003) Fracture-toughening mechanisms responsible for differences in work to fracture of hydrated and dehydrated dentine. Journal of Biomechanics 36, 229–37.
- Karataş E, Gündüz HA, Kırıcı DÖ, Arslan H (2016) Incidence of dentinal cracks after root canal preparation with ProTaper Gold, Profile Vortex, F360, Reciproc and ProTaper Universal instruments. International Endodontic Journal 49, 905–10.
- Kfir A, Elkes D, Pawar A, Weissman A, Tsesis I (2017) Incidence of microcracks in maxillary first premolars after instrumentation with three different mechanize file systems: a comparative ex vivo study. Clinical Oral Investigations 21, 405–11.
- Kishen A (2006) Mechanisms and risk factors for fracture predilection in endodontically treated teeth. Endodontic Topics 13, 57–83.
- Kruzic JJ, Nalla RK, Kinney JH, Ritchie RO (2003) Crack blunting, crack bridging and resistance-curve fracture mechanics in dentin: effect of hydration. Biomaterials 24, 5209–21.
- Liu R, Hou BX, Wesselink PR, Wu MK, Shemesh H (2013) The incidence of root microcracks caused by 3 different single-file systems versus the ProTaper system. Journal of Endodontics 39, 1054–6.
- Pop I, Manoharan A, Zanini F, Tromba G, Patel S, Foschi F (2015) Synchrotron light-based lCT to analyse the presence of dentinal microcracks post-rotary and reciprocating NiTi instrumentation. Clinical Oral Investigations 19, 11–6.
- PradeepKumar AR, Shemesh H, Chang JW et al. (2017) Pre-existing dentinal microcracks in nonendodontically treated teeth: an ex vivo micro-computed tomographic analysis. Journal of Endodontics 43, 896–900.
- Saber SE, Scha€fer E (2016) Incidence of dentinal defects after preparation of severely curved root canals using the Reciproc single-file system with and without prior creation of a glide path. International Endodontic Journal 49, 1057–64.
- Shemesh H, Bier CA, Wu MK, Tanomaru-Filho M, Wesselink PR (2009) The effects of canal preparation and filling on the incidence of dentinal defects. International Endodontic Journal 42, 208–13.
- Shemesh H, Lindtner T, Portoles CA, Zaslansky P (2018) Dehydration induces cracking in root dentin irrespective of instrumentation: a two-dimensional and three-dimensional study. Journal of Endodontics 44, 120–5.
- Yang SF, Rivera EM, Walton RE (1995) Vertical root fracture in nonendodontically treated teeth. Journal of Endodontics 21, 337–9.
- Zuolo ML, De-Deus G, Belladonna FG et al. (2017) Micro-computed tomography assessment of dentinal microcracks after root canal preparation with TRUShape and Self-adjusting file systems. Journal of Endodontics 43, 619–22.