Mesiobuccal and Palatal Interorifice Distance May Predict the Presence of the Second Mesiobuccal Canal in Maxillary Second Molars with Fused Roots
Abstract
Introduction: The aim of this study was to evaluate if the configuration of canal orifices can predict the presence of a second mesiobuccal canal (MB2) in maxillary second molars with fused roots. Methods: Maxillary second molars with fused roots (N = 150) were scanned in a micro–computed tomographic device (pixel size = 9 mm) and evaluated regarding the root fusion type and the incidence of the MB2 canal. The centers of the canal orifices were connected, and the angles formed by the intersection of these lines as well as their distances were measured and statistically compared (1-way analysis of variance). Specimens were then assigned to 2 groups according to the presence (n = 65) or absence (n = 50) of the MB2 canal and compared regarding the angles and interorifice distances using the Welch t test. A binary logistic regression model estimated the association between the interorifice distance, the fusion type, and the presence of the MB2 canal, whereas receiver operating characteristic curve analysis was performed to evaluate the diagnostic abilities of significant variables. The significance level was set at 5%. Results: The most common fusion types were 1 and 6, and MB2 canal incidence was 47.3%. No statistical difference was observed in the angles or interorifice distances among teeth with different fusion types (P ˃ .05). Distances from the mesiobuccal (MB) to the distobuccal and palatal (P) canal orifices as well as the MB and P angles were significantly higher in the presence of an MB2 canal (P ˂ .05). A binary logistic regression model revealed that MB-distobuccal and MB-P distances were significant in the presence of an MB2 canal (P ˂ .05). Receiver operating characteristic analysis indicated that MB-P distance had acceptable diagnostic accuracy for the prediction of MB2 canal presence. Sensitivity (specificity) calculated from the MB-P distances of 4.0, 4.5, and 5.0 mm were 89.2% (36.5%), 76.9% (58.4%), and 44.4% (71.4%), respectively. Conclusions: MB-P interorifice distance is a strong predictor of the presence of the MB2 canal in maxillary second molars with fused roots. (J Endod 2021;47:585–591.)
A predictive and favorable outcome for nonsurgical endodontic treatment requires a comprehensive knowledge about root canal anatomy and its variations. For instance, in maxillary molars, the inability to detect and adequately treat extra canals, such as the second canal of the mesiobuccal root (MB2), has been considered the major cause of failure in root canal therapy. The literature has demonstrated a higher prevalence of the MB2 canal in maxillary first molars compared with second molars, but a significant incidence of complex morphologies and extra canals have also been reported in the latter when roots are fused. Recently, endodontically treated, fused rooted molars were linked to a higher incidence of posttreatment apical periodontitis than nonfused teeth. In clinics, the gold standard imaging technique to evaluate the presence of extra canals is through cone-beam computed tomographic (CBCT) imaging. It would be useful to predict the presence of extra canals based on morphologic aspects of the pulp chamber, considering that intraoral radiography is still the imaging modality of choice for preoperative diagnosis. Previous studies suggested that the distribution of canal orifices on the pulp chamber floor could be used as a factor to predict the presence of extra canals. In nonfused maxillary first molars, a distance ratio greater than 1.26 from the mesiobuccal (MB) and distobuccal (DB) canals to the palatal (P) canal was reported as a predictive factor for MB2 occurrence.
However, to date, no study investigated the association between root canal orifice distribution and the incidence of the MB2 canal in maxillary second molars with fused roots. Therefore, this study aimed to evaluate if the configuration of canal orifices in the pulp chamber floor of maxillary second molars with fused roots can predict the presence of the MB2 canal.
Materials and methods
Sample Selection and Imaging
After the approval of this research protocol by the local ethics board (protocol KAEK-2017-234), 150 extracted maxillary second molars with fused roots free of fractures, deep caries, or restorations were selected from a Turkish subpopulation and stored at 37˚C with 100% humidity. The patients’ sex and age were unknown. The teeth were scanned on a micro– computed tomographic (micro-CT) device (SkyScan 1172; Bruker-microCT, Kontich, Belgium) at 9 mm (pixel size), 100 kV, 100 mA, a 180˚ rotation range with a 0.6˚ step, a camera exposure time of 2200 milliseconds, and a frame average of 1 with an aluminum copper filter. Data reconstruction was performed by NRecon v.1.10.6. software (Bruker-microCT) followed by the alignment of the pulp chamber floor perpendicularly to the long axis of each specimen (DataViewer v.1.5.4.0 software, Bruker-microCT). CTAn v.1.17.7.2 (Bruker-microCT) was used to create 3-dimensional models of the external and internal morphologic structures of the teeth.
Image Analysis
CTVol 2.3.2.0 software (Bruker-microCT) was used to classify the specimens according to the root fusion type (Fig. 1), the number of main root canals, and the incidence of the MB2 canal. Then, in the axial slice corresponding to the pulp chamber floor, the center of each canal orifice was detected and connected.
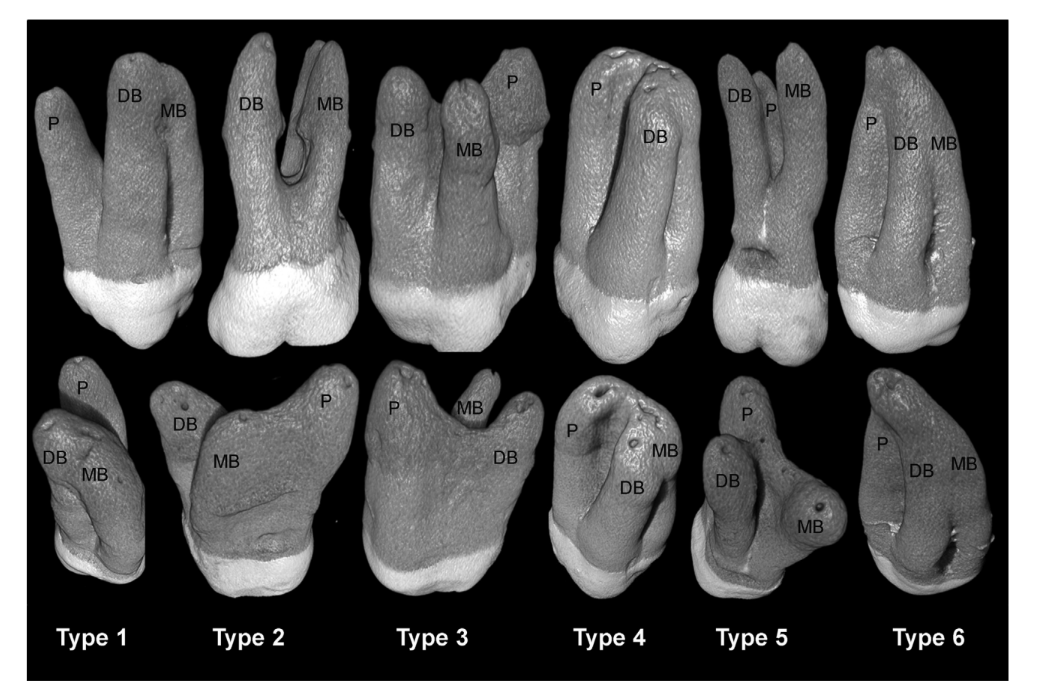
The angles (in degrees) formed by the intersection of these lines (Fig. 2A) as well as the linear distance (in millimeters) between each canal orifice (Fig. 2B) were calculated using AutoCAD software (Autodesk, San Rafael, CA). Additionally, the perpendicular distance between a line connecting the MB and P orifices to the center of the MB2 orifice was measured (red line in Fig. 2B).
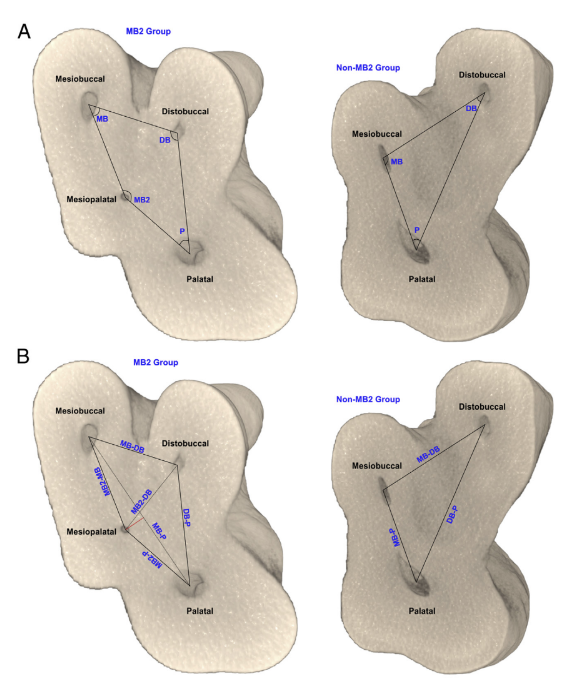
Statistical Analysis
The one-way analysis of variance test was used to compare the interorifice distance among teeth with different root fusion types after excluding specimens with a pulp chamber elongated up to the midroot level (n = 14) and a single root canal (n = 6) orifice at the pulp chamber floor level. After the exclusion of samples with 2 (n = 11) or 5 (n = 4) canals at the pulp chamber floor level, the remaining specimens (n = 115) were further assigned to 2 groups according to the presence (n = 65) or absence (n = 50) of the MB2 canal and statistically compared regarding the interorifice distances and the angles formed by the intersection of lines connecting the centers of the orifices using the Welch t test. A binary logistic regression model was used to estimate the association between 2 independent variables (ie, interorifice distance and root fusion type) and the presence of the MB2 canal (outcome variable). A receiver operating characteristic (ROC) curve was derived to evaluate the significant interorifice distance values and the MB-P/DB-P ratio for the prediction of MB2 canal presence. A confidence interval of 95% was used, and the optimal cutoff values were selected according to sensitivity and specificity values. All analyses were performed using SPSS software (Version 23.0; SPSS Inc, IBM Corp, Armonk, NY) with a significance level set at 5%.
Results
Types 1 (30%) and 6 (19.3%) were the most frequent types of root fusion observed in the maxillary second molars (N = 150), whereas the lowest frequency was type 5 (5.3%). The number of main root canals varied from 1–5, but most of the specimens had 3 (39.3%) or 4 (46.6%) main canals. The presence of the MB2 canal was observed in all types of root fusion in a total of 71 maxillary molars (47.3%). No statistical difference was observed in the interorifice distance when comparing teeth with different fusion types (P ˃ .05) (Table 1).
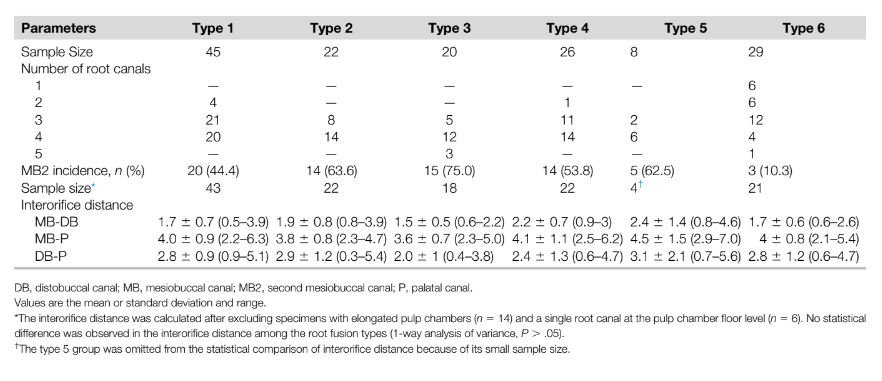
In the comparison of the specimens assigned to 2 groups according to the presence (n = 65) or absence (n = 50) of the MB2 canal, MB-DB and MB-P interorifice distances as well as the MB and P angles were significantly higher in the presence of the MB2 canal (P ˂ .05) (Table 2, Fig. 2C and D).
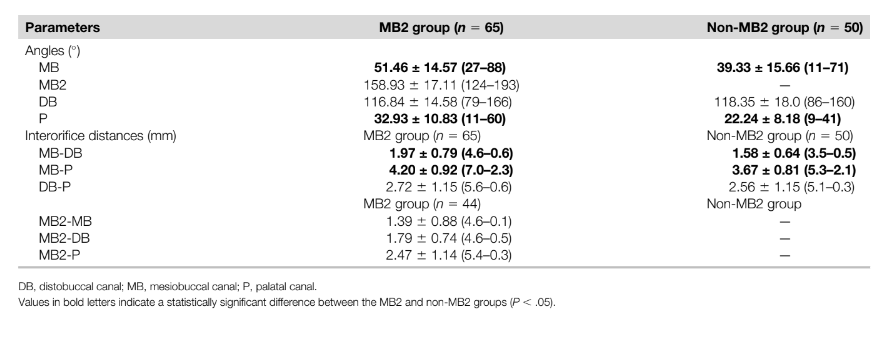
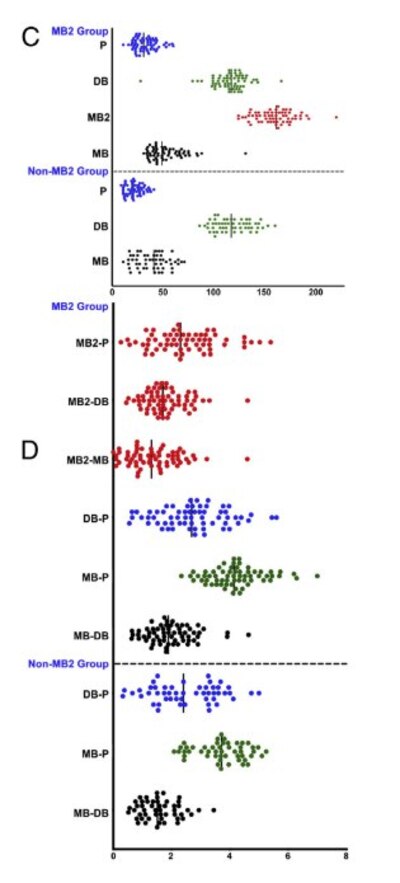
In a binary logistic regression model, MB-DB and MB-P distances showed significant association with MB2 canal presence (P ˂ .05; odds ratio = 1.86 and 2.07, respectively) (Table 3). In the MB2 group, the distance of the MB2 orifice to the MB-P line ranged from 0.19–1.34 mm with a mean value of 0.45 ± 0.32 mm. In 59 molars (90.8%), the center of the MB2 orifice was located mesially to the MB-P interorifice line with a mean distance of 0.48 ± 0.32 mm, whereas in 6 samples (9.2%) it was located slightly distally (0.12 ± 0.05 mm). The ROC curve analysis (Fig. 3) revealed that the MB-P interorifice distance had a greater area under the curve (.72, acceptable) compared with the MB-DB interorifice distance (.63, poor) and the MB-P/DB-P ratio (.52, poor). Thus, the threshold values for the MB-P interorifice distance were further analyzed regarding their sensitivity/specificity for the presence of MB2 canals based on the optimal values observed from 3.52–5.20 mm. The sensitivity (specificity) values calculated from the interorifice distances of 4.0, 4.5, and 5.0 mm were 89.2% (36.5%), 76.9% (58.4%), and 44.4% (71.4%), respectively.
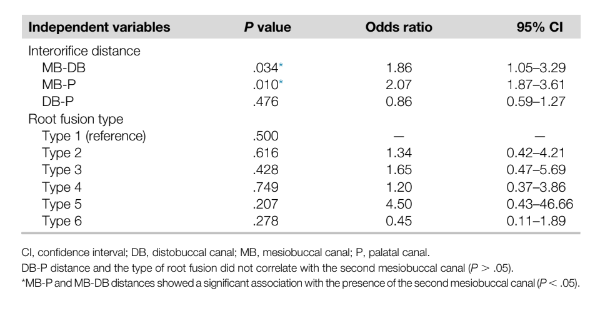
Discussion
Teeth with fused roots can be challenging to treat in clinical practice considering the variations observed in the canal orifice position, which may overstress endodontic instruments, the high incidence of intercanal connections in root with multiple canals, and the presence of a very complex system with multiple foramina at the apical area. Despite its relevance from a clinical perspective, this research topic has not been extensively stressed yet. For instance, until a few years ago, it was believed that C-shaped canals in maxillary molars were a rare anatomic finding, but in 2017, Ordinola-Zapata et al reported this morphology in 22% of maxillary second molars with types 1–5 fused roots. Although unusual, this high incidence was explained by the observational method used (micro-CT imaging) in a large sample size.
Therefore, the current research reports relevant and original data correlating morphologic aspects of the pulp chamber floor and the incidence of the MB2 canal in 150 maxillary second molars with different root fusion types using micro-CT imaging technology as the analytical tool.
In the present study, the highest and lowest incidence of root fusion in maxillary second molars was types 1 (30%) and 5 (5.3%), respectively (Fig. 1, Table 1), which is in agreement with previous micro-CT investigations conducted in Brazilian and Chinese subpopulations. However, the incidence of other fusion types was quite different from those reported in the literature, including a CBCT study that reported type 2 as the most common configuration from a sample of 1335 maxillary second molars in which 25.2% (n = 337) had fused roots. Although these differences might be explained by methodological variations, clinicians must be aware about the high incidence of uncommon morphologies including canal merging, C- shaped morphology, and extra canals in teeth with fused roots. In this study, the highest incidence of the MB2 canal was found in molars with root fusion types 3 (75%), 2 (63%), and 4 (62%), whereas the lowest was type 6 (10%). Nonetheless, notwithstanding differences in the number of major root canals and in the MB2 incidence observed among the root fusion types, no difference was noted in the mean canal orifice distances (Table 1). Therefore, the specimens were combined together into 2 subgroups according to the presence or absence of the MB2 canal and compared.
The rationale behind this study followed the results from a previous CBCT investigation conducted on nonfused maxillary molars that reported an increased likelihood for the presence of the MB2 canal if the MB-P/DB-P interorifice distance ratio was greater than 1.26. This ratio was calculated in the present study, as well as the MB-DB and MB-P interorifice distances using ROC analysis, considering that these distances were significantly greater in the MB2 group than in the non-MB2 group (Table 2). Unlikely to nonfused maxillary molars, in this study, the MB-P distance had a greater area under the curve than the MB-DB distance or the MB- P/DB-P ratio (Fig. 3), indicating it as an acceptable diagnostic factor to predict the presence of the MB2 canal.
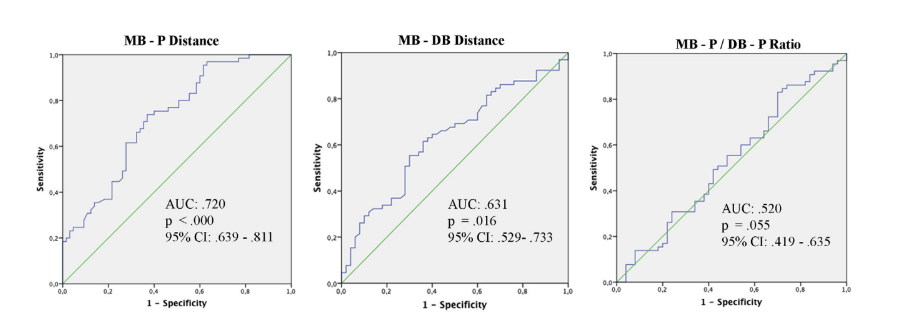
In addition, logistic regression and ROC curve analyses demonstrated that when the MB-P interorifice distance was greater than 4 mm, the presence of the MB2 canal could be highly expected. This information can be very useful during clinical inspection of the pulp chamber floor with the aid of magnification devices in order to locate the MB2 canal without further radiation exposure, which is consistent with the “as low as reasonably achievable” principle advocated by the joint statement of the American Association of Endodontists and the American Academy of Oral and Maxillofacial Radiology on the use of CBCT imaging in endodontics.
In clinics, determining the location of the MB2 canal can be difficult because its orifice might be concealed below a dentin layer formed by tertiary dentin or calcification caused by aging or a pathologic process. To locate the MB2 orifice, it has been recommended to clinicians to explore a hypothetical line connecting the MB1 and P canal orifices 2–3 mm from the former toward the P canal. Although it may be valid in nonfused maxillary molars, applying this orientation to the present sample would allow the identification of only 9.2% of the MB2 orifices once most of them (90.8%) were located ~1.4 mm palatal to the MB1 orifice and from a mean distance of 0.45 ± 0.32 mm (0.19–1.34 mm) mesially to the hypothetical line connecting the MB1 and P orifices.
In this study, the angulations between 2 adjacent orifices were measured to determine the effect of MB2 canal presence and indicated a significant increase in the MB and P angles when the MB2 canal was present. Krasner and Rankow correlated the orifice position with junctions of the chamber floor and walls, which ultimately determines the endodontic access cavity outlines. A similar approach was reported in a CBCT study of nonfused maxillary molars in a Taiwanese population, supporting the modification of the traditional access cavity shape from a triangle to a rhomboidal shape to locate the MB2 orifice more effectively. A significant increase in the MB and P angles caused by the presence of the MB2 canal may indicate that the proposal for the modification of the access cavity design is also valid for maxillary second molars with fused roots.
According to some authors, root fusion occurs as a discontinuity of root maturation or cement deposition between roots by aging. The exact mechanism behind it is still to be determined; however, it has been hypothesized that root canal configuration of molars with fused roots resembles nonfused molars if the fusion is formed by cement deposition, which means that aging may be a relevant factor that influences the internal anatomy. A recent systematic review conducted on CBCT studies also concluded that preoperative factors such as age, sex, and geographic location of the patient might favor clinicians for the anticipation of MB2 canal occurrence in maxillary molars. Therefore, a limitation of the current study was that patients’ ages at the time of teeth extraction were unknown. Further studies are suggested to investigate the effect of aging on the internal anatomy of molar teeth with fused roots.
Conclusions
The MB2 canal was mostly observed in maxillary second molars with root fusion types 1–5. The MB-P interorifice distance is a strong predictor of the presence of the MB2 canal in maxillary second molars with fused roots, mostly when this interorifice distance is greater than 4 mm.
Authors: Cangul Keskin, Ali Kelesx, and Marco Aurelio Versiani
References:
- Setzer FC, Boyer KR, Jeppson JR, et al. Long-term prognosis of endodontically treated teeth: a retrospective analysis of preoperative factors in molars. J Endod 2011;37:21–5.
- Baruwa AO, Martins JN, Meirinhos J, et al. The influence of missed canals on the prevalence of periapical lesions in endodontically treated teeth: a cross-sectional study. J Endod 2020;46:34– 39.e31.
- Karabucak B, Bunes A, Chehoud C, et al. Prevalence of apical periodontitis in endodontically treated premolars and molars with untreated canal: a cone-beam computed tomography study. J Endod 2016;42:538–41.
- Costa F, Pacheco-Yanes J, Siqueira J Jr, et al. Association between missed canals and apical periodontitis. Int Endod J 2019;52:400–6.
- Martins JN, Marques D, Silva EJ, et al. Second mesiobuccal root canal in maxillary molars—a systematic review and meta-analysis of prevalence studies using cone beam computed tomography. Arch Oral Biol 2020;113:104589.
- Ordinola-Zapata R, Martins J, Bramante CM, et al. Morphological evaluation of maxillary second molars with fused roots: a micro-CT study. Int Endod J 2017;50:1192–200.
- Martins JN, Mata A, Marques D, Carame^s J. Prevalence of root fusions and main root canal merging in human upper and lower molars: a cone-beam computed tomography in vivo study. J Endod 2016;42:900–8.
- Bhatt M, Coil J, Chehroudi B, et al. Clinical decision-making and importance of the AAE/AAOMR position statement for CBCT examination in endodontic cases. Int Endod J 2021;54:26–37.
- American Association of Endodontists. Treatment standards. 2020. Available at: https://www. aae.org/specialty/wp-content/uploads/sites/2/2018/04/TreatmentStandards_White paper.pdf. Accessed November 2020.
- Akbarzadeh N, Aminoshariae A, Khalighinejad N, et al. The association between the anatomic landmarks of the pulp chamber floor and the prevalence of middle mesial canals in mandibular first molars: an in vivo analysis. J Endod 2017;43:1797–801.
- Zhang Y, Xu H, Wang D, et al. Assessment of the second mesiobuccal root canal in maxillary first molars: a cone-beam computed tomographic study. J Endod 2017;43:1990–6.
- Zhang Q, Chen H, Fan B, et al. Root and root canal morphology in maxillary second molar with fused root from a native Chinese population. J Endod 2014;40:871–5.
- Vertucci FJ. Root canal morphology and its relationship to endodontic procedures. Endod Topics 2005;10:3–29.
- Fayad MI, Nair M, Levin MD, et al. AAE and AAOMR joint position statement: use of cone beam computed tomography in endodontics 2015 update. Oral Surg Oral Med Oral Pathol Oral Radiol 2015;4:508–12.
- Ibarrola JL, Knowles KI, Ludlow MO, McKinley IB Jr. Factors affecting the negotiability of second mesiobuccal canals in maxillary molars. J Endod 1997;23:236–8.
- Parker J, Mol A, Rivera EM, Tawil P. CBCT uses in clinical endodontics: the effect of CBCT on the ability to locate MB2 canals in maxillary molars. Int Endod J 2017;50:1109–15.
- Ruddle C. Microendodontics: identification and treatment of MBII system. J Calif Dent Assoc 1997;25:313–7.
- Kulid JC, Peters DD. Incidence and configuration of canal systems in the mesiobuccal root of maxillary first and second molars. J Endod 1990;16:311–7.
- Moidu NP, Sharma S, Kumar V, et al. Association between the mesiobuccal canal configuration, interorifice distance, and the corresponding root length of permanent maxillary first molar tooth: a cone-beam computed tomography study. J Endod 2021;47:39–43.
- Zhuk R, Taylor S, Johnson JD, Paranjpe A. Locating the MB2 canal in relation to MB1 in maxillary first molars using CBCT imaging. Aust Endod J 2020;46:184–90.
- Krasner P, Rankow HJ. Anatomy of the pulp-chamber floor. J Endod 2004;30:5–16.
- Su CC, Huang RY, Wu YC, et al. Detection and location of second mesiobuccal canal in permanent maxillary teeth: a cone-beam computed tomography analysis in a Taiwanese population. Arch Oral Biol 2019;98:108–14.
- Al-Fouzan K. C-shaped root canals in mandibular second molars in a Saudi Arabian population. Int Endod J 2002;35:499–504.
- Carlsen O, Alexandersen V, Heitmann T, Jakobsen P. Root canals in one-rooted maxillary second molars. Scand J Dent Res 1992;100:249–56.
To continue learning and get access to all the other articles, log in or create an account